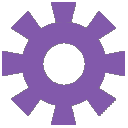
Loading Pathway...
Error: Pathway image not found.
Hide
Pathway Description
Glycerolipid Metabolism
Homo sapiens
Metabolic Pathway
The glycerolipid metabolism pathway describes the synthesis of glycerolipids such as monoacylglycerols (MAGs), diacylglycerols (DAGs), triacylglycerols (TAGs), phosphatidic acids (PAs), and lysophosphatidic acids (LPAs). The process begins with cytoplasmic 3-phosphoglyceric acid (a product of glycolysis). This molecule is dephosphorylated via the enzyme glycerate kinase to produce glyceric acid. Glyceric acid is then transformed to glycerol (via the action of aldehyde dehydrogenase and aldose reductase). The free, cytoplasmic glycerol can then be phosphorylated to glycerol-3-phosphate through the action of glycerol kinase. Glycerol-3-phosphate can then enter the endoplasmic reticulum where glycerol-3-phosphate acyltransferase (GPAT) may combine various acyl-CoA moieties (which donate acyl groups) to form lysophosphatidic (LPA) or phosphatidic acid (PA). The resulting phosphatidic acids can be dephosphorylated via lipid phosphate phosphohydrolase (also known as phosphatidate phosphatase) to produce diacylglycerols (DAGs). The resulting DAGs can be converted into triacylglycerols (TAGs) via the addition of another acyl group (contributed via acyl-CoA) and the action of 1-acyl-sn-glycerol-3-phosphate acyltransferase. Extracellularly, the triacylglycerols (TAGs) can be converted to monoacylglycerols (MAGs) through the action of hepatic triacylglycerol lipase. In addition to this cytoplasmic route of glycerolipid synthesis, another route via mitochondrial synthesis also exists. This route begins with glycerol-3-phosphate, which can be either derived from dihydroxyacetone phosphate (DHAP), a product of glycolysis (usually in the cytoplasm of liver or adipose tissue cells) or from glycerol itself. Glycerol-3-phosphate in the mitochondria is first acylated via acyl-coenzyme A (acyl-CoA) through the action of mitochondrial glycerol-3-phosphate acyltransferase to form lysophosphatidic acid (LPA). Once synthesized, lysophosphatidic acid is then acylated with another molecule of acyl-CoA via the action of 1-acyl-sn-glycerol-3-phosphate acetyltransferase to yield phosphatidic acid. Phosphatidic acid is then dephosphorylated to form diacylglycerol. Specifically, diacylglycerol is formed by the action of phosphatidate phosphatase (also known as lipid phosphate phosphohydrolase) on phosphatidic acid coupled with the release of a phosphate. The phosphatase exists as 3 isozymes. Diacylglycerol is a precursor to triacylglycerol (triglyceride), which is formed in the addition of a third fatty acid to the diacylglycerol by the action of diglyceride acyltransferase. Since diacylglycerol is synthesized via phosphatidic acid, it will usually contain a saturated fatty acid at the C-1 position on the glycerol moiety and an unsaturated fatty acid at the C-2 position. When the body uses stored fat as a source of energy, glycerol and fatty acids are released into the bloodstream. Fatty acids, stored as triglycerides in humans, are an important and a particularly rich source of energy. The energy yield from a gram of fatty acids is approximately 9 kcal/g (39 kJ/g), compared to 4 kcal/g (17 kJ/g) for carbohydrates. Since the hydrocarbon portion of fatty acids is hydrophobic, these molecules can be stored in a relatively anhydrous (water-free) environment. Fatty acids can hold more than six times the amount of energy than sugars on a weight basis. In other words, if you relied on sugars or carbohydrates to store energy, then you would need to carry 67.5 lb (31 kg) of glycogen to have the energy equivalent to 10 lb (5 kg) of fat.
References
Glycerolipid Metabolism References
Lehninger, A.L. Lehninger principles of biochemistry (4th ed.) (2005). New York: W.H Freeman.
Salway, J.G. Metabolism at a glance (3rd ed.) (2004). Alden, Mass.: Blackwell Pub.
Vance, D.E., and Vance, J.E. Biochemistry of lipids, lipoproteins, and membranes (4th ed.) (2002) Amsterdam; Boston: Elsevier.
Zhang P, Reue K: Lipin proteins and glycerolipid metabolism: Roles at the ER membrane and beyond. Biochim Biophys Acta Biomembr. 2017 Sep;1859(9 Pt B):1583-1595. doi: 10.1016/j.bbamem.2017.04.007. Epub 2017 Apr 11.
Pubmed: 28411173
Kiessling V, Crane JM, Tamm LK: Transbilayer effects of raft-like lipid domains in asymmetric planar bilayers measured by single molecule tracking. Biophys J. 2006 Nov 1;91(9):3313-26. doi: 10.1529/biophysj.106.091421. Epub 2006 Aug 11.
Pubmed: 16905614
Rusinol AE, Cui Z, Chen MH, Vance JE: A unique mitochondria-associated membrane fraction from rat liver has a high capacity for lipid synthesis and contains pre-Golgi secretory proteins including nascent lipoproteins. J Biol Chem. 1994 Nov 4;269(44):27494-502.
Pubmed: 7961664
Nagle CA, An J, Shiota M, Torres TP, Cline GW, Liu ZX, Wang S, Catlin RL, Shulman GI, Newgard CB, Coleman RA: Hepatic overexpression of glycerol-sn-3-phosphate acyltransferase 1 in rats causes insulin resistance. J Biol Chem. 2007 May 18;282(20):14807-15. doi: 10.1074/jbc.M611550200. Epub 2007 Mar 27.
Pubmed: 17389595
Helenius J, Ng DT, Marolda CL, Walter P, Valvano MA, Aebi M: Translocation of lipid-linked oligosaccharides across the ER membrane requires Rft1 protein. Nature. 2002 Jan 24;415(6870):447-50. doi: 10.1038/415447a.
Pubmed: 11807558
Alaimo C, Catrein I, Morf L, Marolda CL, Callewaert N, Valvano MA, Feldman MF, Aebi M: Two distinct but interchangeable mechanisms for flipping of lipid-linked oligosaccharides. EMBO J. 2006 Mar 8;25(5):967-76. doi: 10.1038/sj.emboj.7601024. Epub 2006 Feb 23.
Pubmed: 16498400
van Meer G, Voelker DR, Feigenson GW: Membrane lipids: where they are and how they behave. Nat Rev Mol Cell Biol. 2008 Feb;9(2):112-24. doi: 10.1038/nrm2330.
Pubmed: 18216768
Baumann NA, Sullivan DP, Ohvo-Rekila H, Simonot C, Pottekat A, Klaassen Z, Beh CT, Menon AK: Transport of newly synthesized sterol to the sterol-enriched plasma membrane occurs via nonvesicular equilibration. Biochemistry. 2005 Apr 19;44(15):5816-26. doi: 10.1021/bi048296z.
Pubmed: 15823040
Sud M, Fahy E, Cotter D, Brown A, Dennis EA, Glass CK, Merrill AH Jr, Murphy RC, Raetz CR, Russell DW, Subramaniam S: LMSD: LIPID MAPS structure database. Nucleic Acids Res. 2007 Jan;35(Database issue):D527-32. doi: 10.1093/nar/gkl838. Epub 2006 Nov 10.
Pubmed: 17098933
Henry SA, Kohlwein SD, Carman GM: Metabolism and regulation of glycerolipids in the yeast Saccharomyces cerevisiae. Genetics. 2012 Feb;190(2):317-49. doi: 10.1534/genetics.111.130286.
Pubmed: 22345606
Oelkers P, Cromley D, Padamsee M, Billheimer JT, Sturley SL: The DGA1 gene determines a second triglyceride synthetic pathway in yeast. J Biol Chem. 2002 Mar 15;277(11):8877-81. doi: 10.1074/jbc.M111646200. Epub 2001 Dec 18.
Pubmed: 11751875
Gaspar ML, Hofbauer HF, Kohlwein SD, Henry SA: Coordination of storage lipid synthesis and membrane biogenesis: evidence for cross-talk between triacylglycerol metabolism and phosphatidylinositol synthesis. J Biol Chem. 2011 Jan 21;286(3):1696-708. doi: 10.1074/jbc.M110.172296. Epub 2010 Oct 23.
Pubmed: 20972264
Gaspar ML, Aregullin MA, Jesch SA, Henry SA: Inositol induces a profound alteration in the pattern and rate of synthesis and turnover of membrane lipids in Saccharomyces cerevisiae. J Biol Chem. 2006 Aug 11;281(32):22773-85. doi: 10.1074/jbc.M603548200. Epub 2006 Jun 15.
Pubmed: 16777854
Gaspar ML, Jesch SA, Viswanatha R, Antosh AL, Brown WJ, Kohlwein SD, Henry SA: A block in endoplasmic reticulum-to-Golgi trafficking inhibits phospholipid synthesis and induces neutral lipid accumulation. J Biol Chem. 2008 Sep 12;283(37):25735-51. doi: 10.1074/jbc.M802685200. Epub 2008 Jul 9.
Pubmed: 18614533
Highlighted elements will appear in red.
Highlight Compounds
Highlight Proteins
Enter relative concentration values (without units). Elements will be highlighted in a color gradient where red = lowest concentration and green = highest concentration. For the best results, view the pathway in Black and White.
Visualize Compound Data
Visualize Protein Data
Settings