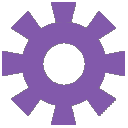
PathWhiz ID | Pathway | Meta Data |
---|---|---|
PW000994 |
disease
Citric Acid Cycle (1st Reaction)Homo sapiens
|
Creator: Guest: Anonymous Created On: July 27, 2015 at 10:47 Last Updated: July 27, 2015 at 10:47 |
PW000993 |
Citric Acid Cycle (need to change)Homo sapiens
|
Creator: Guest: Anonymous Created On: July 23, 2015 at 16:02 Last Updated: July 23, 2015 at 16:02 |
PW000948 |
Citric Acid Cycle 1434490807Homo sapiens
|
Creator: Guest: Anonymous Created On: June 16, 2015 at 15:43 Last Updated: June 16, 2015 at 15:43 |
PW000949 |
Citric Acid Cycle 1434561054Homo sapiens
The citric acid cycle, which is also known as the tricarboxylic acid cycle (TCA cycle) or the Krebs cycle, is a series of enzyme-catalyzed chemical reactions of key importance in all living cells that use oxygen as part of cellular respiration. In eukaryotes, the citric acid cycle occurs in the mitochondrial matrix. The TCA cycle begins with acetyl-CoA transferring its two-carbon acetyl group to the four-carbon acceptor compound (oxaloacetate) to form a six-carbon compound (citrate). The citrate then goes through a series of chemical transformations, losing first one, then a second carboxyl group as CO2. The carbons lost as CO2 originate from what was oxaloacetate, not directly from acetyl-CoA. The carbons donated by acetyl-CoA become part of the oxaloacetate carbon backbone after the first turn of the citric acid cycle. Loss of the acetyl-CoA-donated carbons as CO2 requires several turns of the citric acid cycle. However, because of the role of the citric acid cycle in anabolism, they may not be lost since many TCA cycle intermediates are also used as precursors for the biosynthesis of other molecules. Most of the energy made available by the oxidative steps of the cycle is transferred as energy-rich electrons to NAD+, forming NADH. For each acetyl group that enters the citric acid cycle, three molecules of NADH are produced. At the end of each cycle, the four-carbon oxaloacetate has been regenerated, and the cycle continues - PathWhiz
|
Creator: Guest: Anonymous Created On: June 17, 2015 at 11:11 Last Updated: June 17, 2015 at 11:11 |
PW000950 |
Citric Acid Cycle 1434561204Homo sapiens
The citric acid cycle, which is also known as the tricarboxylic acid cycle (TCA cycle) or the Krebs cycle, is a series of enzyme-catalyzed chemical reactions of key importance in all living cells that use oxygen as part of cellular respiration. In eukaryotes, the citric acid cycle occurs in the mitochondrial matrix. The TCA cycle begins with acetyl-CoA transferring its two-carbon acetyl group to the four-carbon acceptor compound (oxaloacetate) to form a six-carbon compound (citrate). The citrate then goes through a series of chemical transformations, losing first one, then a second carboxyl group as CO2. The carbons lost as CO2 originate from what was oxaloacetate, not directly from acetyl-CoA. The carbons donated by acetyl-CoA become part of the oxaloacetate carbon backbone after the first turn of the citric acid cycle. Loss of the acetyl-CoA-donated carbons as CO2 requires several turns of the citric acid cycle. However, because of the role of the citric acid cycle in anabolism, they may not be lost since many TCA cycle intermediates are also used as precursors for the biosynthesis of other molecules. Most of the energy made available by the oxidative steps of the cycle is transferred as energy-rich electrons to NAD+, forming NADH. For each acetyl group that enters the citric acid cycle, three molecules of NADH are produced. At the end of each cycle, the four-carbon oxaloacetate has been regenerated, and the cycle continues - PathWhiz.
|
Creator: Guest: Anonymous Created On: June 17, 2015 at 11:14 Last Updated: June 17, 2015 at 11:14 |
PW000970 |
Citric Acid Cycle 1434561204Saccharomyces cerevisiae
The citric acid cycle, which is also known as the tricarboxylic acid cycle (TCA cycle) or the Krebs cycle, is a series of enzyme-catalyzed chemical reactions of key importance in all living cells that use oxygen as part of cellular respiration. In eukaryotes, the citric acid cycle occurs in the mitochondrial matrix. The TCA cycle begins with acetyl-CoA transferring its two-carbon acetyl group to the four-carbon acceptor compound (oxaloacetate) to form a six-carbon compound (citrate). The citrate then goes through a series of chemical transformations, losing first one, then a second carboxyl group as CO2. The carbons lost as CO2 originate from what was oxaloacetate, not directly from acetyl-CoA. The carbons donated by acetyl-CoA become part of the oxaloacetate carbon backbone after the first turn of the citric acid cycle. Loss of the acetyl-CoA-donated carbons as CO2 requires several turns of the citric acid cycle. However, because of the role of the citric acid cycle in anabolism, they may not be lost since many TCA cycle intermediates are also used as precursors for the biosynthesis of other molecules. Most of the energy made available by the oxidative steps of the cycle is transferred as energy-rich electrons to NAD+, forming NADH. For each acetyl group that enters the citric acid cycle, three molecules of NADH are produced. At the end of each cycle, the four-carbon oxaloacetate has been regenerated, and the cycle continues - PathWhiz.
|
Creator: Guest: Anonymous Created On: July 09, 2015 at 14:51 Last Updated: July 09, 2015 at 14:51 |
PW123826 |
Citric Acid Cycle 1434561204 1582757517Homo sapiens
|
Creator: Guest: Anonymous Created On: February 26, 2020 at 15:52 Last Updated: February 26, 2020 at 15:52 |
PW123675 |
Citric Acid Cycle 1577989254Saccharomyces cerevisiae
|
Creator: Guest: Anonymous Created On: January 02, 2020 at 11:21 Last Updated: January 02, 2020 at 11:21 |
PW128226 |
Citric Acid Cycle 1691135724Caenorhabditis elegans
The citric acid cycle, which is also known as the tricarboxylic acid cycle (TCA cycle) or the Krebs cycle, is a connected series of enzyme-catalyzed chemical reactions of central importance to all aerobic organisms (i.e. organisms that use oxygen for cellular respiration). The citric acid cycle is named after citrate or citric acid, a tricarboxylic acid that is both consumed and regenerated through this pathway. The citric acid cycle was discovered in 1937 by Hans Adolf Krebs while he worked at the University of Sheffield in England (PMID: 16746382). Krebs received the Nobel Prize for his discovery in 1953. Krebs’ extensive work on this pathway is also why the citric acid or TCA cycle is often referred to as the Krebs cycle. Metabolically, the citric acid cycle allows the release of energy (ultimately in the form of ATP) from carbohydrates, fats, and proteins through the oxidation of acetyl-CoA. The citric acid cycle also produces CO2, the precursors for several amino acids (aspartate, asparagine, glutamine, proline) and NADH – all of which are used in other important metabolic pathways, such as amino acid synthesis and oxidative phosphorylation (OxPhos). The net yield of one “turn” of the TCA cycle in terms of energy-containing compounds is one GTP, one FADH2, and three NADH molecules. The NADH molecules are used in oxidative phosphorylation to generate ATP. In eukaryotes, the citric acid cycle occurs in the mitochondrial matrix. In prokaryotes, the citric acid cycle occurs in the cytoplasm. In eukaryotes, the citric acid or TCA cycle has a total of 10 steps that are mediated by 8 different enzymes. Key to the whole cycle is the availability of acetyl-CoA. One of the primary sources of acetyl-CoA is from the breakdown of glucose (and other sugars) by glycolysis. This process generates pyruvate. Pyruvate is decarboxylated by pyruvate dehydrogenase to generate acetyl-CoA. The citric acid cycle begins with acetyl-CoA transferring its two-carbon acetyl group to the four-carbon acceptor compound (oxaloacetate) to form a six-carbon compound (citrate) through the enzyme citrate synthase. The resulting citrate is then converted to cis-aconitate and then isocitrate via the enzyme aconitase. The resulting isocitrate then combines with NAD+ to form oxalosuccinate and NADH, which is then converted into alpha-ketoglutarate (and CO2) through the action of the enzyme known as isocitrate dehydrogenase. The resulting alpha-ketoglutarate combines with NAD+ and CoA-SH to produce succinyl-CoA, NADH, and CO2. This step is mediated by the enzyme alpha-ketoglutarate dehydrogenase. The resulting succinyl-CoA combines with GDP and organic phosphate to produce succinate, CoA-SH, and GTP. This phosphorylation reaction is performed by succinyl-CoA synthase. The resulting succinate then combines with ubiquinone to produce two compounds, fumarate and ubiquinol through the action of the enzyme succinate dehydrogenase. The resulting fumarate is then hydrated by the enzyme known as fumarase to produce malate. The resulting malate is oxidized via NAD+ to produce oxaloacetate and NADH. This oxidation reaction is performed by malate dehydrogenase. The resulting oxaloacetate can then combine with acetyl-CoA and the TCA reaction cycle begins again. Overall, in the citric acid cycle, the starting six-carbon citrate molecule loses two carboxyl groups as CO2, leading to the production of a four-carbon oxaloacetate. The two-carbon acetyl-CoA that is the “fuel” for the TCA cycle can be generated by several metabolic pathways including glucose metabolism, fatty acid oxidation, and the metabolism of amino acids. The overall reaction for the citric acid cycle is as follows: acetyl-CoA + 3 NAD+ + FAD + GDP + P + 2H2O = CoA-SH + 3NADH + FADH2 + 3H+ + GTP + 2CO2. Many molecules in the citric acid cycle serve as key precursors for other molecules needed by cells. The citrate generated via the citric acid cycle can serve as an intermediate for fatty acid synthesis; alpha-ketoglutarate can serve as a precursor for glutamate, proline, and arginine; oxaloacetate can serve as a precursor for aspartate and asparagine; succinyl-CoA can serve as a precursor for porphyrins; and acetyl-CoA can serve as a precursor fatty acids, cholesterol, vitamin D, and various steroid hormones. There are several variations to the citric acid cycle that are known. Interestingly, most of the variation lies with the step involving succinyl-CoA production or conversion. Humans and other animals have two different types of succinyl-CoA synthetases. One produces GTP from GDP, while the other produces ATP from ADP (PMID: 9765291). On the other hand, plants have a succinyl-CoA synthetase that produces ATP (ADP-forming succinyl-CoA synthetase) (Jones RC, Buchanan BB, Gruissem W. (2000). Biochemistry & molecular biology of plants (1st ed.). Rockville, Md: American Society of Plant Physiologists. ISBN 0-943088-39-9.). In certain acetate-producing bacteria, such as Acetobacter aceti, an enzyme known as succinyl-CoA:acetate CoA-transferase performs this conversion (PMID: 18502856) while in Helicobacter pylori succinyl-CoA:acetoacetate CoA-transferase is responsible for this reaction (PMID: 9325289). The citric acid cycle is regulated in a number of ways but the primary mechanism is by product inhibition. For instance, NADH inhibits pyruvate dehydrogenase, isocitrate dehydrogenase, alpha-ketoglutarate dehydrogenase, and citrate synthase. Acetyl-CoA inhibits pyruvate dehydrogenase, while succinyl-CoA inhibits alpha-ketoglutarate dehydrogenase and citrate synthase. Additionally, ATP inhibits citrate synthase and alpha-ketoglutarate dehydrogenase. Calcium is another important regulator of the citric acid cycle. In particular, it activates pyruvate dehydrogenase phosphatase, which then activates pyruvate dehydrogenase. Calcium also activates isocitrate dehydrogenase and alpha-ketoglutarate dehydrogenase (PMID: 171557).
|
Creator: WishartLab Created On: August 04, 2023 at 01:55 Last Updated: August 04, 2023 at 01:55 |
PW128227 |
Citric Acid Cycle 1691136134Caenorhabditis elegans
The citric acid cycle, which is also known as the tricarboxylic acid cycle (TCA cycle) or the Krebs cycle, is a connected series of enzyme-catalyzed chemical reactions of central importance to all aerobic organisms (i.e. organisms that use oxygen for cellular respiration). The citric acid cycle is named after citrate or citric acid, a tricarboxylic acid that is both consumed and regenerated through this pathway. The citric acid cycle was discovered in 1937 by Hans Adolf Krebs while he worked at the University of Sheffield in England (PMID: 16746382). Krebs received the Nobel Prize for his discovery in 1953. Krebs’ extensive work on this pathway is also why the citric acid or TCA cycle is often referred to as the Krebs cycle. Metabolically, the citric acid cycle allows the release of energy (ultimately in the form of ATP) from carbohydrates, fats, and proteins through the oxidation of acetyl-CoA. The citric acid cycle also produces CO2, the precursors for several amino acids (aspartate, asparagine, glutamine, proline) and NADH – all of which are used in other important metabolic pathways, such as amino acid synthesis and oxidative phosphorylation (OxPhos). The net yield of one “turn” of the TCA cycle in terms of energy-containing compounds is one GTP, one FADH2, and three NADH molecules. The NADH molecules are used in oxidative phosphorylation to generate ATP. In eukaryotes, the citric acid cycle occurs in the mitochondrial matrix. In prokaryotes, the citric acid cycle occurs in the cytoplasm. In eukaryotes, the citric acid or TCA cycle has a total of 10 steps that are mediated by 8 different enzymes. Key to the whole cycle is the availability of acetyl-CoA. One of the primary sources of acetyl-CoA is from the breakdown of glucose (and other sugars) by glycolysis. This process generates pyruvate. Pyruvate is decarboxylated by pyruvate dehydrogenase to generate acetyl-CoA. The citric acid cycle begins with acetyl-CoA transferring its two-carbon acetyl group to the four-carbon acceptor compound (oxaloacetate) to form a six-carbon compound (citrate) through the enzyme citrate synthase. The resulting citrate is then converted to cis-aconitate and then isocitrate via the enzyme aconitase. The resulting isocitrate then combines with NAD+ to form oxalosuccinate and NADH, which is then converted into alpha-ketoglutarate (and CO2) through the action of the enzyme known as isocitrate dehydrogenase. The resulting alpha-ketoglutarate combines with NAD+ and CoA-SH to produce succinyl-CoA, NADH, and CO2. This step is mediated by the enzyme alpha-ketoglutarate dehydrogenase. The resulting succinyl-CoA combines with GDP and organic phosphate to produce succinate, CoA-SH, and GTP. This phosphorylation reaction is performed by succinyl-CoA synthase. The resulting succinate then combines with ubiquinone to produce two compounds, fumarate and ubiquinol through the action of the enzyme succinate dehydrogenase. The resulting fumarate is then hydrated by the enzyme known as fumarase to produce malate. The resulting malate is oxidized via NAD+ to produce oxaloacetate and NADH. This oxidation reaction is performed by malate dehydrogenase. The resulting oxaloacetate can then combine with acetyl-CoA and the TCA reaction cycle begins again. Overall, in the citric acid cycle, the starting six-carbon citrate molecule loses two carboxyl groups as CO2, leading to the production of a four-carbon oxaloacetate. The two-carbon acetyl-CoA that is the “fuel” for the TCA cycle can be generated by several metabolic pathways including glucose metabolism, fatty acid oxidation, and the metabolism of amino acids. The overall reaction for the citric acid cycle is as follows: acetyl-CoA + 3 NAD+ + FAD + GDP + P + 2H2O = CoA-SH + 3NADH + FADH2 + 3H+ + GTP + 2CO2. Many molecules in the citric acid cycle serve as key precursors for other molecules needed by cells. The citrate generated via the citric acid cycle can serve as an intermediate for fatty acid synthesis; alpha-ketoglutarate can serve as a precursor for glutamate, proline, and arginine; oxaloacetate can serve as a precursor for aspartate and asparagine; succinyl-CoA can serve as a precursor for porphyrins; and acetyl-CoA can serve as a precursor fatty acids, cholesterol, vitamin D, and various steroid hormones. There are several variations to the citric acid cycle that are known. Interestingly, most of the variation lies with the step involving succinyl-CoA production or conversion. Humans and other animals have two different types of succinyl-CoA synthetases. One produces GTP from GDP, while the other produces ATP from ADP (PMID: 9765291). On the other hand, plants have a succinyl-CoA synthetase that produces ATP (ADP-forming succinyl-CoA synthetase) (Jones RC, Buchanan BB, Gruissem W. (2000). Biochemistry & molecular biology of plants (1st ed.). Rockville, Md: American Society of Plant Physiologists. ISBN 0-943088-39-9.). In certain acetate-producing bacteria, such as Acetobacter aceti, an enzyme known as succinyl-CoA:acetate CoA-transferase performs this conversion (PMID: 18502856) while in Helicobacter pylori succinyl-CoA:acetoacetate CoA-transferase is responsible for this reaction (PMID: 9325289). The citric acid cycle is regulated in a number of ways but the primary mechanism is by product inhibition. For instance, NADH inhibits pyruvate dehydrogenase, isocitrate dehydrogenase, alpha-ketoglutarate dehydrogenase, and citrate synthase. Acetyl-CoA inhibits pyruvate dehydrogenase, while succinyl-CoA inhibits alpha-ketoglutarate dehydrogenase and citrate synthase. Additionally, ATP inhibits citrate synthase and alpha-ketoglutarate dehydrogenase. Calcium is another important regulator of the citric acid cycle. In particular, it activates pyruvate dehydrogenase phosphatase, which then activates pyruvate dehydrogenase. Calcium also activates isocitrate dehydrogenase and alpha-ketoglutarate dehydrogenase (PMID: 171557).
|
Creator: WishartLab Created On: August 04, 2023 at 02:02 Last Updated: August 04, 2023 at 02:02 |