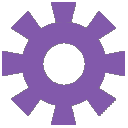
PathWhiz ID | Pathway | Meta Data |
---|---|---|
PW088538 |
Mitochondrial Beta-Oxidation of Short Chain Saturated Fatty AcidsCaenorhabditis elegans
Beta-oxidation is the major degradative pathway for fatty acid esters in humans. Fatty acids and their CoA esters are found throughout the body, playing roles such as components of cellular lipids, regulators of enzymes and membrane channels, ligands for nuclear receptors, precursor molecules for hormones, and signalling molecules. Beta-oxidation occurs in the peroxisomes and mitochondria, the latter of which is depicted here. Whether beta-oxidation starts in the mitochondria or the peroxisome depends on the length of the fatty acid. Medium to long chain fatty acids go directly to the mitochondria, whereas very long chain fatty acids (>22 carbons) may be first metabolized down to octanyl-CoA in the peroxisomes and then transported to the mitochondria for the remainder of the oxidation. Beta-oxidation begins with fatty acids first being activated by an acyl-coenzyme A synthetase. This process uses ATP to produce a reactive fatty acyl adenylate which then reacts with coenzyme A to produce a fatty acyl-CoA. Short and medium chain fatty acids can enter the mitochondria directly via diffusion where they are activated in the mitochondrial matrix by acyl-coenzyme A synthetases. Long chain fatty acids must be activated in the outer mitochondrial membrane then transported as a carnatine complex into the mitochondria. A double bond is formed between C-2 and C-3 to produce trans-Δ2-enoyl-CoA which is catalyzed by acyl-CoA-dehydrogenases in the mitochondria. Enoyl CoA hydratase then hydrates the double bond between C-2 and C-3 to produce a L-beta-hydroxyacyl CoA which then has its hydroxyl group converted to a keto group to produce beta-ketoacyl CoA. Finally, the beta-ketoacyl CoA is cleaved by beta-ketothiolase and a thiol group is inserted between C-2 and C-3 to reduce the acyl-CoA and produce acetyl-CoA. Acetyl-CoA can then enter the citric acid cycle.
|
Creator: Ana Marcu Created On: August 10, 2018 at 18:22 Last Updated: August 10, 2018 at 18:22 |
PW000524 |
disease
Mitochondrial Complex II DeficiencyHomo sapiens
Mitochondrial complex II deficiency, which is also known as CII deficiency, is a rare form of an inherited inborn error of metabolism (IEM). CII deficiency is an autosomal recessive disorder that arises from mutations in the succinate dehydrogenase (SDH) genes (SDHA, SDHB, SDHC and SDHD). These genes code for the mitochondrial enzyme known as succinate dehydrogenase, a multicomponent, membrane-bound enzyme, which is also known as SDH, succinate-coenzyme Q reductase (SQR), or respiratory complex II. SDH is found in the inner mitochondrial membrane and catalyzes the oxidation of succinate to fumarate with the reduction of ubiquinone to ubiquinol. SDH or complex II is assembled via the action of two assembly factors (SDHAF1 and SDHAF2). Mutations in SDHA and SDHAF1 are most commonly found in patients with CII deficiency. Because complex II is found in the mitochondria, CII deficiency is technically considered a mitochondrial disease. CII deficiency accounts for between 2%-23% of all respiratory chain deficiency diagnoses. The signs and symptoms of mitochondrial complex II deficiency can vary greatly from severe life-threatening symptoms in infancy to muscle disease beginning in adulthood. The symptoms are very much dependent on the mutations to the SDH components. SDHA gene mutations cause myoclonic seizures and Leigh’s syndrome, a severe neurological disorder that is characterized by progressive loss of mental and movement abilities (psychomotor regression) and typically results in death within 1-2 years. SDHB gene mutations can cause leukodystrophy which affects the myelin sheath, the material surrounding and protecting nerve cells. Damage to the myelin sheath slows down or blocks messages between the brain and the rest of the body, which leads to problems with movement, speech, vision, hearing, and mental and physical development. SDHAF1 gene mutations can cause severe progressive leukoencephalopathy, which is characterized by the degeneration of the white matter of the brain. Interestingly, complex II deficiency gene mutation carriers may be at an increased risk for certain cancers.
|
Creator: WishartLab Created On: August 29, 2013 at 10:39 Last Updated: August 29, 2013 at 10:39 |
PW122092 |
disease
Mitochondrial Complex II DeficiencyRattus norvegicus
Mitochondrial complex II deficiency, which is also known as CII deficiency, is a rare form of an inherited inborn error of metabolism (IEM). CII deficiency is an autosomal recessive disorder that arises from mutations in the succinate dehydrogenase (SDH) genes (SDHA, SDHB, SDHC and SDHD). These genes code for the mitochondrial enzyme known as succinate dehydrogenase, a multicomponent, membrane-bound enzyme, which is also known as SDH, succinate-coenzyme Q reductase (SQR), or respiratory complex II. SDH is found in the inner mitochondrial membrane and catalyzes the oxidation of succinate to fumarate with the reduction of ubiquinone to ubiquinol. SDH or complex II is assembled via the action of two assembly factors (SDHAF1 and SDHAF2). Mutations in SDHA and SDHAF1 are most commonly found in patients with CII deficiency. Because complex II is found in the mitochondria, CII deficiency is technically considered a mitochondrial disease. CII deficiency accounts for between 2%-23% of all respiratory chain deficiency diagnoses. The signs and symptoms of mitochondrial complex II deficiency can vary greatly from severe life-threatening symptoms in infancy to muscle disease beginning in adulthood. The symptoms are very much dependent on the mutations to the SDH components. SDHA gene mutations cause myoclonic seizures and Leigh’s syndrome, a severe neurological disorder that is characterized by progressive loss of mental and movement abilities (psychomotor regression) and typically results in death within 1-2 years. SDHB gene mutations can cause leukodystrophy which affects the myelin sheath, the material surrounding and protecting nerve cells. Damage to the myelin sheath slows down or blocks messages between the brain and the rest of the body, which leads to problems with movement, speech, vision, hearing, and mental and physical development. SDHAF1 gene mutations can cause severe progressive leukoencephalopathy, which is characterized by the degeneration of the white matter of the brain. Interestingly, complex II deficiency gene mutation carriers may be at an increased risk for certain cancers.
|
Creator: Ana Marcu Created On: September 10, 2018 at 15:52 Last Updated: September 10, 2018 at 15:52 |
PW127321 |
disease
Mitochondrial Complex II DeficiencyHomo sapiens
Mitochondrial complex II deficiency, which is also known as CII deficiency, is a rare form of an inherited inborn error of metabolism (IEM). CII deficiency is an autosomal recessive disorder that arises from mutations in the succinate dehydrogenase (SDH) genes (SDHA, SDHB, SDHC and SDHD). These genes code for the mitochondrial enzyme known as succinate dehydrogenase, a multicomponent, membrane-bound enzyme, which is also known as SDH, succinate-coenzyme Q reductase (SQR), or respiratory complex II. SDH is found in the inner mitochondrial membrane and catalyzes the oxidation of succinate to fumarate with the reduction of ubiquinone to ubiquinol. SDH or complex II is assembled via the action of two assembly factors (SDHAF1 and SDHAF2). Mutations in SDHA and SDHAF1 are most commonly found in patients with CII deficiency. Because complex II is found in the mitochondria, CII deficiency is technically considered a mitochondrial disease. CII deficiency accounts for between 2%-23% of all respiratory chain deficiency diagnoses. The signs and symptoms of mitochondrial complex II deficiency can vary greatly from severe life-threatening symptoms in infancy to muscle disease beginning in adulthood. The symptoms are very much dependent on the mutations to the SDH components. SDHA gene mutations cause myoclonic seizures and Leigh’s syndrome, a severe neurological disorder that is characterized by progressive loss of mental and movement abilities (psychomotor regression) and typically results in death within 1-2 years. SDHB gene mutations can cause leukodystrophy which affects the myelin sheath, the material surrounding and protecting nerve cells. Damage to the myelin sheath slows down or blocks messages between the brain and the rest of the body, which leads to problems with movement, speech, vision, hearing, and mental and physical development. SDHAF1 gene mutations can cause severe progressive leukoencephalopathy, which is characterized by the degeneration of the white matter of the brain. Interestingly, complex II deficiency gene mutation carriers may be at an increased risk for certain cancers.
|
Creator: Ray Kruger Created On: December 08, 2022 at 15:50 Last Updated: December 08, 2022 at 15:50 |
PW121868 |
disease
Mitochondrial Complex II DeficiencyMus musculus
Mitochondrial complex II deficiency, which is also known as CII deficiency, is a rare form of an inherited inborn error of metabolism (IEM). CII deficiency is an autosomal recessive disorder that arises from mutations in the succinate dehydrogenase (SDH) genes (SDHA, SDHB, SDHC and SDHD). These genes code for the mitochondrial enzyme known as succinate dehydrogenase, a multicomponent, membrane-bound enzyme, which is also known as SDH, succinate-coenzyme Q reductase (SQR), or respiratory complex II. SDH is found in the inner mitochondrial membrane and catalyzes the oxidation of succinate to fumarate with the reduction of ubiquinone to ubiquinol. SDH or complex II is assembled via the action of two assembly factors (SDHAF1 and SDHAF2). Mutations in SDHA and SDHAF1 are most commonly found in patients with CII deficiency. Because complex II is found in the mitochondria, CII deficiency is technically considered a mitochondrial disease. CII deficiency accounts for between 2%-23% of all respiratory chain deficiency diagnoses. The signs and symptoms of mitochondrial complex II deficiency can vary greatly from severe life-threatening symptoms in infancy to muscle disease beginning in adulthood. The symptoms are very much dependent on the mutations to the SDH components. SDHA gene mutations cause myoclonic seizures and Leigh’s syndrome, a severe neurological disorder that is characterized by progressive loss of mental and movement abilities (psychomotor regression) and typically results in death within 1-2 years. SDHB gene mutations can cause leukodystrophy which affects the myelin sheath, the material surrounding and protecting nerve cells. Damage to the myelin sheath slows down or blocks messages between the brain and the rest of the body, which leads to problems with movement, speech, vision, hearing, and mental and physical development. SDHAF1 gene mutations can cause severe progressive leukoencephalopathy, which is characterized by the degeneration of the white matter of the brain. Interestingly, complex II deficiency gene mutation carriers may be at an increased risk for certain cancers.
|
Creator: Ana Marcu Created On: September 10, 2018 at 15:50 Last Updated: September 10, 2018 at 15:50 |
PW122081 |
disease
Mitochondrial DNA Depletion SyndromeRattus norvegicus
Mitochondrial DNA depletion syndromes are a group of autosomal recessive disorders. They are characterized by decreased levels of mitochondrial DNA (mtDNA), resulting in decreased energy production. Mitochondrial DNA depletion syndrome-3 is caused by a mutation in the gene coding for deoxyguanosine kinase (DGUOK). DGUOK aids in maintaining the levels of available deoxyguanosine triphosphate (dGTP), thus the deficiency of DGUOK impairs the synthesis of dGTP. Symptoms of DGUOK deficiency can arise in the form of a multi-system disease in neonates or an isolated hepatic disease in infancy, with the former being more common. The most common cause of death in both forms is progressive hepative disease.
|
Creator: Ana Marcu Created On: September 10, 2018 at 15:52 Last Updated: September 10, 2018 at 15:52 |
PW121857 |
disease
Mitochondrial DNA Depletion SyndromeMus musculus
Mitochondrial DNA depletion syndromes are a group of autosomal recessive disorders. They are characterized by decreased levels of mitochondrial DNA (mtDNA), resulting in decreased energy production. Mitochondrial DNA depletion syndrome-3 is caused by a mutation in the gene coding for deoxyguanosine kinase (DGUOK). DGUOK aids in maintaining the levels of available deoxyguanosine triphosphate (dGTP), thus the deficiency of DGUOK impairs the synthesis of dGTP. Symptoms of DGUOK deficiency can arise in the form of a multi-system disease in neonates or an isolated hepatic disease in infancy, with the former being more common. The most common cause of death in both forms is progressive hepative disease.
|
Creator: Ana Marcu Created On: September 10, 2018 at 15:50 Last Updated: September 10, 2018 at 15:50 |
PW127301 |
disease
Mitochondrial DNA Depletion Syndrome-3Homo sapiens
Mitochondrial DNA depletion syndromes are a group of autosomal recessive disorders. They are characterized by decreased levels of mitochondrial DNA (mtDNA), resulting in decreased energy production. Mitochondrial DNA depletion syndrome-3 is caused by a mutation in the gene coding for deoxyguanosine kinase (DGUOK). DGUOK aids in maintaining the levels of available deoxyguanosine triphosphate (dGTP), thus the deficiency of DGUOK impairs the synthesis of dGTP. Symptoms of DGUOK deficiency can arise in the form of a multi-system disease in neonates or an isolated hepatic disease in infancy, with the former being more common. The most common cause of death in both forms is progressive hepative disease.
|
Creator: Ray Kruger Created On: December 05, 2022 at 10:04 Last Updated: December 05, 2022 at 10:04 |
PW000512 |
disease
Mitochondrial DNA Depletion Syndrome-3Homo sapiens
Mitochondrial DNA depletion syndromes are a group of autosomal recessive disorders. They are characterized by decreased levels of mitochondrial DNA (mtDNA), resulting in decreased energy production. Mitochondrial DNA depletion syndrome-3 is caused by a mutation in the gene coding for deoxyguanosine kinase (DGUOK). DGUOK aids in maintaining the levels of available deoxyguanosine triphosphate (dGTP), thus the deficiency of DGUOK impairs the synthesis of dGTP. Symptoms of DGUOK deficiency can arise in the form of a multi-system disease in neonates or an isolated hepatic disease in infancy, with the former being more common. The most common cause of death in both forms is progressive hepative disease.
|
Creator: WishartLab Created On: August 29, 2013 at 10:39 Last Updated: August 29, 2013 at 10:39 |
PW127433 |
Mitochondrial Electron Transport ChainPlasmodium falciparum
The electron transport chain in mitochondria leads to the transport of hydrogen ions across the inner membrane of the mitochndria, and this proton gradient is eventually used in the production of ATP. Electrons travel down a chain of electron carriers in the inner mitochondrial membrane, ending with oxygen.
The outer membrane of the mitochondrion is permeable to ions and other small molecules and nothing in this pathway requires a specific transporter to enter into the intermembrane space. However, the inner membrane is only permeable to water, oxygen and carbon dioxide, and all other molecules, including protons, require transport proteins. Phosphate is able to enter the mitochondrial matrix via the glucose-6-phosphate translocase, and ADP is able to enter the matrix as ATP leaves it via the ADP/ATP translocase 1 protein.
Electrons donated by NADH can enter the electron transport chain as NADH dehydrogenase, known as complex I, facilitates their transfer to ubiquinone, also known as coenzyme Q10. As this occurs, the coenzyme Q10 becomes reduced to form ubiquinol, and protons are pumped from the intermembrane space to the matrix.
Lower energy electrons can also be donated to complex II, which includes succinate dehydrogenase and contains FAD. These electrons move from succinic acid to the FAD in the enzyme complex, and then to coenzyme Q10, which is reduced to ubiquinol. Throughout this, succinic acid from the citric acid cycle is converted to fumaric acid, which then returns to the citric acid cycle. This step, unlike the others in the electron transport chain, does not result in any protons being pumped from the matrix to the intermembrane space.
Regardless of which complex moved the electrons to coenzyme Q10, the cytochrome b-c1 complex, also known as complex III, catalyzes the movement of electrons from ubiquinol to cytochrome c, oxidizing ubiquinol to ubiquinone and reducing cytochrome c. This process also leads to the pumping of hydrogen ions into the intermembrane space.
Finally, the transfer of electrons from the reduced cytochrome c is catalyzed by cytochrome c oxidase, also known as complex IV of the electron transport chain. This reaction oxidizes cytochrome c for further electron transport, and transfers the electrons to oxygen, forming molecules of water. This reaction also allows protons to be pumped across the membrane.
The proton gradient that is built up through the electron transport chain allows protons to flow through the ATP synthase proteins in the mitochondrial inner membrane, providing the energy required to synthesize ATP from ADP.
|
Creator: Ray Kruger Created On: February 02, 2023 at 11:34 Last Updated: February 02, 2023 at 11:34 |