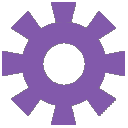
PathWhiz ID | Pathway | Meta Data |
---|---|---|
PW145079 |
drug action
Physostigmine Drug Metabolism Action PathwayHomo sapiens
|
Creator: Ray Kruger Created On: October 07, 2023 at 15:02 Last Updated: October 07, 2023 at 15:02 |
PW176398 |
Physostigmine Predicted Metabolism PathwayHomo sapiens
Metabolites of Physostigmine are predicted with biotransformer.
|
Creator: Omolola Created On: December 07, 2023 at 16:48 Last Updated: December 07, 2023 at 16:48 |
PW088409 |
Phytanic Acid Peroxisomal OxidationDrosophila melanogaster
Phytanic acid, a branched chain fatty acid, is an important component of fatty acid intake, occuring in meat, fish and dairy products. Due to its methylation, it cannot be a substrate for acyl-CoA dehydrogenase and cannot enter the mitochondrial beta oxidation pathway. Phytanic acid is instead activated to its CoA ester form by a CoA synthetase to phytanoyl-CoA, where it can begin the first cycle of alpha oxidation. Phytanoyl-CoA is a substrate for a specific alpha-hydroxylase (Phytanoyl-CoA hydroxylase), which adds a hydroxyl group to the α-carbon of phytanic acid, creating the 19-carbon homologue, pristanic acid. Pristanic acid then undergoes further metabolism through beta oxidation.
|
Creator: Ana Marcu Created On: August 10, 2018 at 15:51 Last Updated: August 10, 2018 at 15:51 |
PW064633 |
Phytanic Acid Peroxisomal OxidationMus musculus
Phytanic acid, a branched chain fatty acid, is an important component of fatty acid intake, occuring in meat, fish and dairy products. Due to its methylation, it cannot be a substrate for acyl-CoA dehydrogenase and cannot enter the mitochondrial beta oxidation pathway. Phytanic acid is instead activated to its CoA ester form by a CoA synthetase to phytanoyl-CoA, where it can begin the first cycle of alpha oxidation. Phytanoyl-CoA is a substrate for a specific alpha-hydroxylase (Phytanoyl-CoA hydroxylase), which adds a hydroxyl group to the α-carbon of phytanic acid, creating the 19-carbon homologue, pristanic acid. Pristanic acid then undergoes further metabolism through beta oxidation.
|
Creator: Carin Li Created On: January 21, 2018 at 22:38 Last Updated: January 21, 2018 at 22:38 |
PW088331 |
Phytanic Acid Peroxisomal OxidationRattus norvegicus
Phytanic acid, a branched chain fatty acid, is an important component of fatty acid intake, occuring in meat, fish and dairy products. Due to its methylation, it cannot be a substrate for acyl-CoA dehydrogenase and cannot enter the mitochondrial beta oxidation pathway. Phytanic acid is instead activated to its CoA ester form by a CoA synthetase to phytanoyl-CoA, where it can begin the first cycle of alpha oxidation. Phytanoyl-CoA is a substrate for a specific alpha-hydroxylase (Phytanoyl-CoA hydroxylase), which adds a hydroxyl group to the α-carbon of phytanic acid, creating the 19-carbon homologue, pristanic acid. Pristanic acid then undergoes further metabolism through beta oxidation.
|
Creator: Ana Marcu Created On: August 10, 2018 at 13:49 Last Updated: August 10, 2018 at 13:49 |
PW088464 |
Phytanic Acid Peroxisomal OxidationCaenorhabditis elegans
Phytanic acid, a branched chain fatty acid, is an important component of fatty acid intake, occuring in meat, fish and dairy products. Due to its methylation, it cannot be a substrate for acyl-CoA dehydrogenase and cannot enter the mitochondrial beta oxidation pathway. Phytanic acid is instead activated to its CoA ester form by a CoA synthetase to phytanoyl-CoA, where it can begin the first cycle of alpha oxidation. Phytanoyl-CoA is a substrate for a specific alpha-hydroxylase (Phytanoyl-CoA hydroxylase), which adds a hydroxyl group to the α-carbon of phytanic acid, creating the 19-carbon homologue, pristanic acid. Pristanic acid then undergoes further metabolism through beta oxidation.
|
Creator: Ana Marcu Created On: August 10, 2018 at 17:09 Last Updated: August 10, 2018 at 17:09 |
PW088236 |
Phytanic Acid Peroxisomal OxidationBos taurus
Phytanic acid, a branched chain fatty acid, is an important component of fatty acid intake, occuring in meat, fish and dairy products. Due to its methylation, it cannot be a substrate for acyl-CoA dehydrogenase and cannot enter the mitochondrial beta oxidation pathway. Phytanic acid is instead activated to its CoA ester form by a CoA synthetase to phytanoyl-CoA, where it can begin the first cycle of alpha oxidation. Phytanoyl-CoA is a substrate for a specific alpha-hydroxylase (Phytanoyl-CoA hydroxylase), which adds a hydroxyl group to the α-carbon of phytanic acid, creating the 19-carbon homologue, pristanic acid. Pristanic acid then undergoes further metabolism through beta oxidation.
|
Creator: Ana Marcu Created On: August 10, 2018 at 11:32 Last Updated: August 10, 2018 at 11:32 |
PW000041 |
Phytanic Acid Peroxisomal OxidationHomo sapiens
Phytanic acid, a branched chain fatty acid, is an important component of fatty acid intake, occuring in meat, fish and dairy products. Due to its methylation, it cannot be a substrate for acyl-CoA dehydrogenase and cannot enter the mitochondrial beta oxidation pathway. Phytanic acid is instead activated to its CoA ester form by a CoA synthetase to phytanoyl-CoA, where it can begin the first cycle of alpha oxidation. Phytanoyl-CoA is a substrate for a specific alpha-hydroxylase (Phytanoyl-CoA hydroxylase), which adds a hydroxyl group to the α-carbon of phytanic acid, creating the 19-carbon homologue, pristanic acid. Pristanic acid then undergoes further metabolism through beta oxidation.
|
Creator: WishartLab Created On: August 01, 2013 at 13:54 Last Updated: August 01, 2013 at 13:54 |
PW012889 |
Phytate BiosynthesisArabidopsis thaliana
Phytate biosynthesis is a pathway that occurs in the cytosol by which myo-inositol becomes D-myo-inositol (1,3,4)-trisphosphate becomes phytate, the principal storage form of phosphorus in many plant tissues . First, myo-inositol-1,3,4-trisphosphate 5/6-kinase uses ATP to catalyze the conversion of D-myo-inositol (1,3,4)-trisphosphate into either D-myo-inositol (1,3,4,6)-tetrakisphosphate or D-myo-inositol (1,3,4,5)-tetrakisphosphate. It requires magnesium ion as a cofactor. Second, inositol polyphosphate multiple-kinase uses ATP to catalyze the conversion of either D-myo-inositol (1,3,4,6)-tetrakisphosphate or D-myo-inositol (1,3,4,5)-tetrakisphosphate into D-myo-inositol 1,3,4,5,6-pentakisphosphate. Third, polyphosphate 2-kinase uses ATP to catalyze the conversion of D-myo-inositol 1,3,4,5,6-pentakisphosphate into phytate. It requires zinc ion as a cofactor.
|
Creator: Carin Li Created On: February 21, 2017 at 21:28 Last Updated: February 21, 2017 at 21:28 |
PW064767 |
signaling
PI3KHomo sapiens
Activation of different types of RTKs leads to the activation of PI3K. This causes the conversion of PIP2 to PIP3 at the plasma membrane. Inactive AKT translocate from the cytoplasm to the plasma membrane where PIP3 binds AKT, leading to activation of AKT by phosphorylation by PDK1 and mTOR. The arrows and the bars represent activation and inhibition of the following proteins, respectively.
|
Creator: Pascal Created On: June 18, 2018 at 05:25 Last Updated: June 18, 2018 at 05:25 |