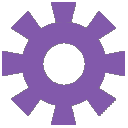
PathWhiz ID | Pathway | Meta Data |
---|---|---|
PW121841 |
disease
Transaldolase DeficiencyMus musculus
Transaldolase deficiency, also known as Eyaid syndrome or TALDO deficiency, is a desease caused by homozygous or compound heterozygous mutations in the TALDO1 gene that encodes for transaldolase. The mutation found in one study was a base pair deletion leading to a premature truncation of the protein, preventing its activity in the cell. Other mutations reported in other studies include other deletions or homozygous base pair substitutions that cause a misfolded and non-functional protein.
Transaldolase is an enzyme that reversibly converts D-erythrose 4-phosphate and fructose 6-phosphate to D-sedoheptulose 7-phosphate and D-glyceraldehyde 3-phosphate, as a part of the pentose phosphate pathway.
Almost all affected patients show hepatosplenomegaly, liver dysfunction, low counts for all blood cell types, cardiac defects, and come from consanguinous families. They also show dysmorphic features, including a triangular face, low set ears, and a wide mouth with thin lips. Other signs include abnormal concentrations of polyols in urine and plasma, as well as ribose-, xylulose-, and ribulose-5-phosphate being elevated in urine.
|
Creator: Ana Marcu Created On: September 10, 2018 at 15:50 Last Updated: September 10, 2018 at 15:50 |
PW127220 |
disease
Transaldolase DeficiencyHomo sapiens
Transaldolase deficiency, also known as Eyaid syndrome or TALDO deficiency, is a desease caused by homozygous or compound heterozygous mutations in the TALDO1 gene that encodes for transaldolase. The mutation found in one study was a base pair deletion leading to a premature truncation of the protein, preventing its activity in the cell. Other mutations reported in other studies include other deletions or homozygous base pair substitutions that cause a misfolded and non-functional protein. Transaldolase is an enzyme that reversibly converts D-erythrose 4-phosphate and fructose 6-phosphate to D-sedoheptulose 7-phosphate and D-glyceraldehyde 3-phosphate, as a part of the pentose phosphate pathway. Almost all affected patients show hepatosplenomegaly, liver dysfunction, low counts for all blood cell types, cardiac defects, and come from consanguinous families. They also show dysmorphic features, including a triangular face, low set ears, and a wide mouth with thin lips. Other signs include abnormal concentrations of polyols in urine and plasma, as well as ribose-, xylulose-, and ribulose-5-phosphate being elevated in urine.
|
Creator: Ray Kruger Created On: November 14, 2022 at 16:55 Last Updated: November 14, 2022 at 16:55 |
PW000455 |
Transcription/TranslationHomo sapiens
Transcription is the mechanism by which a template strand of DNA is utilized by specific RNA polymerases to generate one of the three different classifications of RNA. These 3 RNA classes include messenger RNAs (mRNAs), transfer RNAs (tRNAs) and ribosomal RNAs (rRNAs). mRNAs are the genetic coding templates used by the translational machinery to determine the order of amino acids incorporated into an elongating polypeptide in the process of translation. tRNAs form covalent attachments to individual amino acids and recognize the encoded sequences of the mRNAs to allow correct insertion of amino acids into the elongating polypeptide chain. rRNAs are assembled, together with numerous ribosomal proteins, to form the ribosomes. All RNA polymerases are dependent upon a DNA template in order to synthesize RNA. The resultant RNA is, therefore, complimentary to the template strand of the DNA duplex and identical to the non-template strand. In eukaryotic cells there are 3 distinct classes of RNA polymerase, RNA polymerase (pol) I, II and III. Each polymerase is responsible for the synthesis of a different class of RNA. Once the mRNA transcript is generated, it is available for translation through the action of the ribosome and activated amino-acid tRNA carriers. For protein synthesis, a succession of tRNA molecules charged with appropriate amino acids have to be brought together with an mRNA molecule and matched up by base-pairing through their anti-codons with each of its successive codons. The amino acids then have to be linked together to extend the growing protein chain, and the tRNAs, relieved of their burdens, have to be released. This whole complex of processes is carried out by a giant multimolecular machine, the ribosome, formed of two main chains of RNA, called ribosomal RNA (rRNA), and more than 50 different proteins. The synthesis of proteins is known as translation. Translation occurs in the cytoplasm where the ribosomes are located. Ribosomes are made of a small and large subunit which surrounds the mRNA. In translation, messenger RNA (mRNA) is decoded to produce a specific polypeptide according to the rules specified by the trinucleotide genetic code. This uses an mRNA sequence as a template to guide the synthesis of a chain of amino acids that form a protein. Translation proceeds in four phases: activation, initiation, elongation and termination (all describing the growth of the amino acid chain, or polypeptide that is the product of translation). In activation, the correct amino acid is joined to the correct transfer RNA (tRNA). While this is not technically a step in translation, it is required for translation to proceed. The amino acid is joined by its carboxyl group to the 3’ OH of the tRNA by an ester bond. When the tRNA has an amino acid linked to it, it is termed “charged”. Initiation involves the small subunit of the ribosome binding to 5’ end of mRNA with the help of initiation factors (IF), other proteins that assist the process. Elongation occurs when the next aminoacyl-tRNA (charged tRNA) in line binds to the ribosome along with GTP and an elongation factor. Termination of the polypeptide happens when the A site of the ribosome faces a stop codon (UAA, UAG, or UGA). When this happens, no tRNA can recognize it, but releasing factor can recognize nonsense codons and causes the release of the polypeptide chain.
|
Creator: WishartLab Created On: August 22, 2013 at 11:51 Last Updated: August 22, 2013 at 11:51 |
PW088511 |
Transfer of Acetyl Groups into MitochondriaCaenorhabditis elegans
Acetyl-CoA is an important molecule, which is precursor to HMG CoA, which is a vital component in cholesterol and ketone synthesis. Acetyl CoA participates in the biosynthesis of fatty acids and sterols, in the oxidation of fatty acids and in the metabolism of many amino acids. It also acts as a biological acetylating agent. Acetyl-CoA is made in the mitochondria by metabolizing fatty acids, and the oxidation of pyruvate of acetyl-CoA. When the body has an excess of ATP, the energy in acetyl-Coa can be stored in the form of fatty acids. Acetyl-CoA must cross the mitochondrial membrane to the cytosol, where fatty acid synthesis takes place. Acetyl-CoA is combined with oxalacetic acid by the enzyme citrate synthase, creating citric acid. Citric acid is then transported out of the mitochondria, to the cytosol, where the enzyme citrate lyase converts citric acid back into acetyl-CoA and oxalacetic acid. Malate dehydrogenase reduces oxalacetic acid to malate, which then is either transported back into the mitochondria by the malate-alpha ketoglutarate transporter or oxidized to pyruvate by malic enzyme. Pyruvate can then be transported back into the mitochondria and undergo decarboxylation into oxalacetic acid. Malate can also be used to create NADH by the conversion of malate to oxalacetic acid by malate dehydrogenase.
|
Creator: Ana Marcu Created On: August 10, 2018 at 18:00 Last Updated: August 10, 2018 at 18:00 |
PW064663 |
Transfer of Acetyl Groups into MitochondriaMus musculus
Acetyl-CoA is an important molecule, which is precursor to HMG CoA, which is a vital component in cholesterol and ketone synthesis. Acetyl CoA participates in the biosynthesis of fatty acids and sterols, in the oxidation of fatty acids and in the metabolism of many amino acids. It also acts as a biological acetylating agent. Acetyl-CoA is made in the mitochondria by metabolizing fatty acids, and the oxidation of pyruvate of acetyl-CoA. When the body has an excess of ATP, the energy in acetyl-Coa can be stored in the form of fatty acids. Acetyl-CoA must cross the mitochondrial membrane to the cytosol, where fatty acid synthesis takes place. Acetyl-CoA is combined with oxalacetic acid by the enzyme citrate synthase, creating citric acid. Citric acid is then transported out of the mitochondria, to the cytosol, where the enzyme citrate lyase converts citric acid back into acetyl-CoA and oxalacetic acid. Malate dehydrogenase reduces oxalacetic acid to malate, which then is either transported back into the mitochondria by the malate-alpha ketoglutarate transporter or oxidized to pyruvate by malic enzyme. Pyruvate can then be transported back into the mitochondria and undergo decarboxylation into oxalacetic acid. Malate can also be used to create NADH by the conversion of malate to oxalacetic acid by malate dehydrogenase.
|
Creator: Carin Li Created On: January 22, 2018 at 00:02 Last Updated: January 22, 2018 at 00:02 |
PW088366 |
Transfer of Acetyl Groups into MitochondriaRattus norvegicus
Acetyl-CoA is an important molecule, which is precursor to HMG CoA, which is a vital component in cholesterol and ketone synthesis. Acetyl CoA participates in the biosynthesis of fatty acids and sterols, in the oxidation of fatty acids and in the metabolism of many amino acids. It also acts as a biological acetylating agent. Acetyl-CoA is made in the mitochondria by metabolizing fatty acids, and the oxidation of pyruvate of acetyl-CoA. When the body has an excess of ATP, the energy in acetyl-Coa can be stored in the form of fatty acids. Acetyl-CoA must cross the mitochondrial membrane to the cytosol, where fatty acid synthesis takes place. Acetyl-CoA is combined with oxalacetic acid by the enzyme citrate synthase, creating citric acid. Citric acid is then transported out of the mitochondria, to the cytosol, where the enzyme citrate lyase converts citric acid back into acetyl-CoA and oxalacetic acid. Malate dehydrogenase reduces oxalacetic acid to malate, which then is either transported back into the mitochondria by the malate-alpha ketoglutarate transporter or oxidized to pyruvate by malic enzyme. Pyruvate can then be transported back into the mitochondria and undergo decarboxylation into oxalacetic acid. Malate can also be used to create NADH by the conversion of malate to oxalacetic acid by malate dehydrogenase.
|
Creator: Ana Marcu Created On: August 10, 2018 at 15:04 Last Updated: August 10, 2018 at 15:04 |
PW088425 |
Transfer of Acetyl Groups into MitochondriaDrosophila melanogaster
Acetyl-CoA is an important molecule, which is precursor to HMG CoA, which is a vital component in cholesterol and ketone synthesis. Acetyl CoA participates in the biosynthesis of fatty acids and sterols, in the oxidation of fatty acids and in the metabolism of many amino acids. It also acts as a biological acetylating agent. Acetyl-CoA is made in the mitochondria by metabolizing fatty acids, and the oxidation of pyruvate of acetyl-CoA. When the body has an excess of ATP, the energy in acetyl-Coa can be stored in the form of fatty acids. Acetyl-CoA must cross the mitochondrial membrane to the cytosol, where fatty acid synthesis takes place. Acetyl-CoA is combined with oxalacetic acid by the enzyme citrate synthase, creating citric acid. Citric acid is then transported out of the mitochondria, to the cytosol, where the enzyme citrate lyase converts citric acid back into acetyl-CoA and oxalacetic acid. Malate dehydrogenase reduces oxalacetic acid to malate, which then is either transported back into the mitochondria by the malate-alpha ketoglutarate transporter or oxidized to pyruvate by malic enzyme. Pyruvate can then be transported back into the mitochondria and undergo decarboxylation into oxalacetic acid. Malate can also be used to create NADH by the conversion of malate to oxalacetic acid by malate dehydrogenase.
|
Creator: Ana Marcu Created On: August 10, 2018 at 16:21 Last Updated: August 10, 2018 at 16:21 |
PW088273 |
Transfer of Acetyl Groups into MitochondriaBos taurus
Acetyl-CoA is an important molecule, which is precursor to HMG CoA, which is a vital component in cholesterol and ketone synthesis. Acetyl CoA participates in the biosynthesis of fatty acids and sterols, in the oxidation of fatty acids and in the metabolism of many amino acids. It also acts as a biological acetylating agent. Acetyl-CoA is made in the mitochondria by metabolizing fatty acids, and the oxidation of pyruvate of acetyl-CoA. When the body has an excess of ATP, the energy in acetyl-Coa can be stored in the form of fatty acids. Acetyl-CoA must cross the mitochondrial membrane to the cytosol, where fatty acid synthesis takes place. Acetyl-CoA is combined with oxalacetic acid by the enzyme citrate synthase, creating citric acid. Citric acid is then transported out of the mitochondria, to the cytosol, where the enzyme citrate lyase converts citric acid back into acetyl-CoA and oxalacetic acid. Malate dehydrogenase reduces oxalacetic acid to malate, which then is either transported back into the mitochondria by the malate-alpha ketoglutarate transporter or oxidized to pyruvate by malic enzyme. Pyruvate can then be transported back into the mitochondria and undergo decarboxylation into oxalacetic acid. Malate can also be used to create NADH by the conversion of malate to oxalacetic acid by malate dehydrogenase.
|
Creator: Ana Marcu Created On: August 10, 2018 at 12:52 Last Updated: August 10, 2018 at 12:52 |
PW000154 |
Transfer of Acetyl Groups into MitochondriaHomo sapiens
Acetyl-CoA is an important molecule, which is precursor to HMG CoA, which is a vital component in cholesterol and ketone synthesis. Acetyl CoA participates in the biosynthesis of fatty acids and sterols, in the oxidation of fatty acids and in the metabolism of many amino acids. It also acts as a biological acetylating agent. Acetyl-CoA is made in the mitochondria by metabolizing fatty acids, and the oxidation of pyruvate of acetyl-CoA. When the body has an excess of ATP, the energy in acetyl-Coa can be stored in the form of fatty acids. Acetyl-CoA must cross the mitochondrial membrane to the cytosol, where fatty acid synthesis takes place. Acetyl-CoA is combined with oxalacetic acid by the enzyme citrate synthase, creating citric acid. Citric acid is then transported out of the mitochondria, to the cytosol, where the enzyme citrate lyase converts citric acid back into acetyl-CoA and oxalacetic acid. Malate dehydrogenase reduces oxalacetic acid to malate, which then is either transported back into the mitochondria by the malate-alpha ketoglutarate transporter or oxidized to pyruvate by malic enzyme. Pyruvate can then be transported back into the mitochondria and undergo decarboxylation into oxalacetic acid. Malate can also be used to create NADH by the conversion of malate to oxalacetic acid by malate dehydrogenase.
|
Creator: WishartLab Created On: August 19, 2013 at 12:04 Last Updated: August 19, 2013 at 12:04 |
PW128276 |
drug action
Tranylcypromine Amine Oxidase Norepinephrine Antidepressant Action PathwayHomo sapiens
Tranylcypromine is a non-hydrazine monoamine oxidase inhibitor belonging to the class of antidepressants called MAOIs. This drug is indicated in the treatment of major depression, dysthymic disorder, and atypical depression. It also is useful in panic and phobic disorders. The monoamine oxidase is an enzyme that catalyzes the oxidative deamination of many amines like serotonin, norepinephrine, epinephrine, and dopamine. There are 2 isoforms of this protein: A and B. The first one is found in cells located in the periphery and breakdown serotonin, norepinephrine, epinephrine, dopamine, and tyramine. The second one, the B isoform, breakdowns phenylethylamine, norepinephrine, epinephrine, dopamine, and tyramine. This isoform is found in the extracellular tissues and mostly in the brain. The mechanism of action of the MAOIs is still not determined, it is thought that they act by increasing free serotonin and norepinephrine concentrations and/or by altering the concentrations of other amines in the CNS. MAO A inhibition is thought to be more relevant to antidepressant activity than the inhibition caused by MAO B. Selective MAO B inhibitors have no antidepressant effects. An overdose of this drug will result in insomnia, restlessness, and anxiety. Hypotension, dizziness, weakness, and drowsiness may occur, progressing in severe cases to extreme dizziness and shock. This drug is administered as an oral tablet.
|
Creator: Daphnee Created On: August 14, 2023 at 15:56 Last Updated: August 14, 2023 at 15:56 |