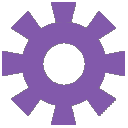
Loading Pathway...
Error: Pathway image not found.
Hide
Pathway Description
Citric Acid Cycle
Bos taurus
Category:
Metabolite Pathway
Sub-Category:
Metabolic
Created: 2018-08-10
Last Updated: 2019-08-16
The citric acid cycle, which is also known as the tricarboxylic acid cycle (TCA cycle) or the Krebs cycle, is a connected series of enzyme-catalyzed chemical reactions of central importance to all aerobic organisms (i.e. organisms that use oxygen for cellular respiration). The citric acid cycle is named after citrate or citric acid, a tricarboxylic acid that is both consumed and regenerated through this pathway. The citric acid cycle was discovered in 1937 by Hans Adolf Krebs while he worked at the University of Sheffield in England (PMID: 16746382). Krebs received the Nobel Prize for his discovery in 1953. Krebs’ extensive work on this pathway is also why the citric acid or TCA cycle is often referred to as the Krebs cycle. Metabolically, the citric acid cycle allows the release of energy (ultimately in the form of ATP) from carbohydrates, fats, and proteins through the oxidation of acetyl-CoA. The citric acid cycle also produces CO2, the precursors for several amino acids (aspartate, asparagine, glutamine, proline) and NADH – all of which are used in other important metabolic pathways, such as amino acid synthesis and oxidative phosphorylation (OxPhos). The net yield of one “turn” of the TCA cycle in terms of energy-containing compounds is one GTP, one FADH2, and three NADH molecules. The NADH molecules are used in oxidative phosphorylation to generate ATP. In eukaryotes, the citric acid cycle occurs in the mitochondrial matrix. In prokaryotes, the citric acid cycle occurs in the cytoplasm. In eukaryotes, the citric acid or TCA cycle has a total of 10 steps that are mediated by 8 different enzymes. Key to the whole cycle is the availability of acetyl-CoA. One of the primary sources of acetyl-CoA is from the breakdown of glucose (and other sugars) by glycolysis. This process generates pyruvate. Pyruvate is decarboxylated by pyruvate dehydrogenase to generate acetyl-CoA. The citric acid cycle begins with acetyl-CoA transferring its two-carbon acetyl group to the four-carbon acceptor compound (oxaloacetate) to form a six-carbon compound (citrate) through the enzyme citrate synthase. The resulting citrate is then converted to cis-aconitate and then isocitrate via the enzyme aconitase. The resulting isocitrate then combines with NAD+ to form oxalosuccinate and NADH, which is then converted into alpha-ketoglutarate (and CO2) through the action of the enzyme known as isocitrate dehydrogenase. The resulting alpha-ketoglutarate combines with NAD+ and CoA-SH to produce succinyl-CoA, NADH, and CO2. This step is mediated by the enzyme alpha-ketoglutarate dehydrogenase. The resulting succinyl-CoA combines with GDP and organic phosphate to produce succinate, CoA-SH, and GTP. This phosphorylation reaction is performed by succinyl-CoA synthase. The resulting succinate then combines with ubiquinone to produce two compounds, fumarate and ubiquinol through the action of the enzyme succinate dehydrogenase. The resulting fumarate is then hydrated by the enzyme known as fumarase to produce malate. The resulting malate is oxidized via NAD+ to produce oxaloacetate and NADH. This oxidation reaction is performed by malate dehydrogenase. The resulting oxaloacetate can then combine with acetyl-CoA and the TCA reaction cycle begins again. Overall, in the citric acid cycle, the starting six-carbon citrate molecule loses two carboxyl groups as CO2, leading to the production of a four-carbon oxaloacetate. The two-carbon acetyl-CoA that is the “fuel” for the TCA cycle can be generated by several metabolic pathways including glucose metabolism, fatty acid oxidation, and the metabolism of amino acids. The overall reaction for the citric acid cycle is as follows: acetyl-CoA + 3 NAD+ + FAD + GDP + P + 2H2O = CoA-SH + 3NADH + FADH2 + 3H+ + GTP + 2CO2. Many molecules in the citric acid cycle serve as key precursors for other molecules needed by cells. The citrate generated via the citric acid cycle can serve as an intermediate for fatty acid synthesis; alpha-ketoglutarate can serve as a precursor for glutamate, proline, and arginine; oxaloacetate can serve as a precursor for aspartate and asparagine; succinyl-CoA can serve as a precursor for porphyrins; and acetyl-CoA can serve as a precursor fatty acids, cholesterol, vitamin D, and various steroid hormones. There are several variations to the citric acid cycle that are known. Interestingly, most of the variation lies with the step involving succinyl-CoA production or conversion. Humans and other animals have two different types of succinyl-CoA synthetases. One produces GTP from GDP, while the other produces ATP from ADP (PMID: 9765291). On the other hand, plants have a succinyl-CoA synthetase that produces ATP (ADP-forming succinyl-CoA synthetase) (Jones RC, Buchanan BB, Gruissem W. (2000). Biochemistry & molecular biology of plants (1st ed.). Rockville, Md: American Society of Plant Physiologists. ISBN 0-943088-39-9.). In certain acetate-producing bacteria, such as Acetobacter aceti, an enzyme known as succinyl-CoA:acetate CoA-transferase performs this conversion (PMID: 18502856) while in Helicobacter pylori succinyl-CoA:acetoacetate CoA-transferase is responsible for this reaction (PMID: 9325289). The citric acid cycle is regulated in a number of ways but the primary mechanism is by product inhibition. For instance, NADH inhibits pyruvate dehydrogenase, isocitrate dehydrogenase, alpha-ketoglutarate dehydrogenase, and citrate synthase. Acetyl-CoA inhibits pyruvate dehydrogenase, while succinyl-CoA inhibits alpha-ketoglutarate dehydrogenase and citrate synthase. Additionally, ATP inhibits citrate synthase and alpha-ketoglutarate dehydrogenase. Calcium is another important regulator of the citric acid cycle. In particular, it activates pyruvate dehydrogenase phosphatase, which then activates pyruvate dehydrogenase. Calcium also activates isocitrate dehydrogenase and alpha-ketoglutarate dehydrogenase (PMID: 171557).
References
Citric Acid Cycle References
Harhay GP, Sonstegard TS, Keele JW, Heaton MP, Clawson ML, Snelling WM, Wiedmann RT, Van Tassell CP, Smith TP: Characterization of 954 bovine full-CDS cDNA sequences. BMC Genomics. 2005 Nov 23;6:166. doi: 10.1186/1471-2164-6-166.
Pubmed: 16305752
Ho L, Javed AA, Pepin RA, Thekkumkara TJ, Raefsky C, Mole JE, Caliendo AM, Kwon MS, Kerr DS, Patel MS: Identification of a cDNA clone for the beta-subunit of the pyruvate dehydrogenase component of human pyruvate dehydrogenase complex. Biochem Biophys Res Commun. 1988 Feb 15;150(3):904-8. doi: 10.1016/0006-291x(88)90714-0.
Pubmed: 2829898
Rahmatullah M, Gopalakrishnan S, Andrews PC, Chang CL, Radke GA, Roche TE: Subunit associations in the mammalian pyruvate dehydrogenase complex. Structure and role of protein X and the pyruvate dehydrogenase component binding domain of the dihydrolipoyl transacetylase component. J Biol Chem. 1989 Feb 5;264(4):2221-7.
Pubmed: 2914903
Neagle J, De Marcucci O, Dunbar B, Lindsay JG: Component X of mammalian pyruvate dehydrogenase complex: structural and functional relationship to the lipoate acetyltransferase (E2) component. FEBS Lett. 1989 Aug 14;253(1-2):11-5. doi: 10.1016/0014-5793(89)80919-6.
Pubmed: 2759236
Rice JE, Dunbar B, Lindsay JG: Sequences directing dihydrolipoamide dehydrogenase (E3) binding are located on the 2-oxoglutarate dehydrogenase (E1) component of the mammalian 2-oxoglutarate dehydrogenase multienzyme complex. EMBO J. 1992 Sep;11(9):3229-35.
Pubmed: 1505515
Zimin AV, Delcher AL, Florea L, Kelley DR, Schatz MC, Puiu D, Hanrahan F, Pertea G, Van Tassell CP, Sonstegard TS, Marcais G, Roberts M, Subramanian P, Yorke JA, Salzberg SL: A whole-genome assembly of the domestic cow, Bos taurus. Genome Biol. 2009;10(4):R42. doi: 10.1186/gb-2009-10-4-r42. Epub 2009 Apr 24.
Pubmed: 19393038
Du J, Zhou Y, Su X, Yu JJ, Khan S, Jiang H, Kim J, Woo J, Kim JH, Choi BH, He B, Chen W, Zhang S, Cerione RA, Auwerx J, Hao Q, Lin H: Sirt5 is a NAD-dependent protein lysine demalonylase and desuccinylase. Science. 2011 Nov 11;334(6057):806-9. doi: 10.1126/science.1207861.
Pubmed: 22076378
Zeng Y, Weiss C, Yao TT, Huang J, Siconolfi-Baez L, Hsu P, Rushbrook JI: Isocitrate dehydrogenase from bovine heart: primary structure of subunit 3/4. Biochem J. 1995 Sep 1;310 ( Pt 2):507-16. doi: 10.1042/bj3100507.
Pubmed: 7654189
Rushbrook JI, Harvey RA: Nicotinamide adenine dinucleotide dependent isocitrate dehydrogenase from beef heart: subunit heterogeneity and enzyme dissociation. Biochemistry. 1978 Dec 12;17(25):5339-46. doi: 10.1021/bi00618a003.
Pubmed: 215197
Weiss C, Zeng Y, Huang J, Sobocka MB, Rushbrook JI: Bovine NAD+-dependent isocitrate dehydrogenase: alternative splicing and tissue-dependent expression of subunit 1. Biochemistry. 2000 Feb 22;39(7):1807-16. doi: 10.1021/bi991691i.
Pubmed: 10677231
Bradford AP, Aitken A, Beg F, Cook KG, Yeaman SJ: Amino acid sequence surrounding the lipoic acid cofactor of bovine kidney 2-oxoglutarate dehydrogenase complex. FEBS Lett. 1987 Sep 28;222(1):211-4. doi: 10.1016/0014-5793(87)80221-1.
Pubmed: 3115829
Agca C, Bidwell CA, Donkin SS: Cloning of bovine pyruvate carboxylase and 5' untranslated region variants. Anim Biotechnol. 2004 May;15(1):47-66. doi: 10.1081/ABIO-120037897.
Pubmed: 15248600
Sonstegard TS, Kappes SM: Mapping of the SDHA locus to bovine chromosome 20. Anim Genet. 1999 Dec;30(6):473.
Pubmed: 10612251
Birch-Machin MA, Farnsworth L, Ackrell BA, Cochran B, Jackson S, Bindoff LA, Aitken A, Diamond AG, Turnbull DM: The sequence of the flavoprotein subunit of bovine heart succinate dehydrogenase. J Biol Chem. 1992 Jun 5;267(16):11553-8.
Pubmed: 1375942
Morris AA, Farnsworth L, Ackrell BA, Turnbull DM, Birch-Machin MA: The cDNA sequence of the flavoprotein subunit of human heart succinate dehydrogenase. Biochim Biophys Acta. 1994 Mar 29;1185(1):125-8. doi: 10.1016/0005-2728(94)90203-8.
Pubmed: 8142412
Cochran B, Capaldi RA, Ackrell BA: The cDNA sequence of beef heart CII-3, a membrane-intrinsic subunit of succinate-ubiquinone oxidoreductase. Biochim Biophys Acta. 1994 Nov 1;1188(1-2):162-6. doi: 10.1016/0005-2728(94)90035-3.
Pubmed: 7947903
Yu L, Wei YY, Usui S, Yu CA: Cytochrome b560 (QPs1) of mitochondrial succinate-ubiquinone reductase. Immunochemistry, cloning, and nucleotide sequencing. J Biol Chem. 1992 Dec 5;267(34):24508-15.
Pubmed: 1447196
Shenoy SK, Yu L, Yu CA: The smallest membrane anchoring subunit (QPs3) of bovine heart mitochondrial succinate-ubiquinone reductase. Cloning, sequencing, topology, and Q-binding domain. J Biol Chem. 1997 Jul 11;272(28):17867-72. doi: 10.1074/jbc.272.28.17867.
Pubmed: 9211943
Plank DW, Howard JB: Identification of the reactive sulfhydryl and sequences of cysteinyl-tryptic peptides from beef heart aconitase. J Biol Chem. 1988 Jun 15;263(17):8184-9.
Pubmed: 3372519
Plank DW, Kennedy MC, Beinert H, Howard JB: Cysteine labeling studies of beef heart aconitase containing a 4Fe, a cubane 3Fe, or a linear 3Fe cluster. J Biol Chem. 1989 Dec 5;264(34):20385-93.
Pubmed: 2511202
Lauble H, Kennedy MC, Beinert H, Stout CD: Crystal structures of aconitase with isocitrate and nitroisocitrate bound. Biochemistry. 1992 Mar 17;31(10):2735-48. doi: 10.1021/bi00125a014.
Pubmed: 1547214
This pathway was propagated using PathWhiz -
Pon, A. et al. Pathways with PathWhiz (2015) Nucleic Acids Res. 43(Web Server issue): W552–W559.
Propagated from SMP0000057
Highlighted elements will appear in red.
Highlight Compounds
Highlight Proteins
Enter relative concentration values (without units). Elements will be highlighted in a color gradient where red = lowest concentration and green = highest concentration. For the best results, view the pathway in Black and White.
Visualize Compound Data
Visualize Protein Data
Downloads
Settings