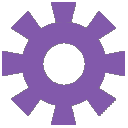
Loading Pathway...
Error: Pathway image not found.
Hide
Pathway Description
Amino Sugar and Nucleotide Sugar Metabolism III
Escherichia coli HS
Category:
Metabolite Pathway
Sub-Category:
Metabolic
Created: 2025-01-21
Last Updated: 2025-01-21
The synthesis of amino sugars and nucleotide sugars starts with the phosphorylation of N-Acetylmuramic acid (MurNac) through its transport from the periplasmic space to the cytoplasm. Once in the cytoplasm, MurNac and water undergo a reversible reaction catalyzed by N-acetylmuramic acid 6-phosphate etherase, producing a D-lactic acid and N-Acetyl-D-Glucosamine 6-phosphate. This latter compound can also be introduced into the cytoplasm through a phosphorylating PTS permase in the inner membrane that allows for the transport of N-Acetyl-D-glucosamine from the periplasmic space. N-Acetyl-D-Glucosamine 6-phosphate can also be obtained from chitin dependent reactions. Chitin is hydrated through a bifunctional chitinase to produce chitobiose. This in turn gets hydrated by a beta-hexosaminidase to produce N-acetyl-D-glucosamine. The latter undergoes an atp dependent phosphorylation leading to the production of N-Acetyl-D-Glucosamine 6-phosphate. N-Acetyl-D-Glucosamine 6-phosphate is then be deacetylated in order to produce Glucosamine 6-phosphate through a N-acetylglucosamine-6-phosphate deacetylase. This compound can either be isomerized or deaminated into Beta-D-fructofuranose 6-phosphate through a glucosamine-fructose-6-phosphate aminotransferase and a glucosamine-6-phosphate deaminase respectively.
Glucosamine 6-phosphate undergoes a reversible reaction to glucosamine 1 phosphate through a phosphoglucosamine mutase. This compound is then acetylated through a bifunctional protein glmU to produce a N-Acetyl glucosamine 1-phosphate.
N-Acetyl glucosamine 1-phosphate enters the nucleotide sugar synthesis by reacting with UTP and hydrogen ion through a bifunctional protein glmU releasing pyrophosphate and a Uridine diphosphate-N-acetylglucosamine.This compound can either be isomerized into a UDP-N-acetyl-D-mannosamine or undergo a reaction with phosphoenolpyruvic acid through UDP-N-acetylglucosamine 1-carboxyvinyltransferase releasing a phosphate and a UDP-N-Acetyl-alpha-D-glucosamine-enolpyruvate.
UDP-N-acetyl-D-mannosamine undergoes a NAD dependent dehydrogenation through a UDP-N-acetyl-D-mannosamine dehydrogenase, releasing NADH, a hydrogen ion and a UDP-N-Acetyl-alpha-D-mannosaminuronate, This compound is then used in the production of enterobacterial common antigens.
UDP-N-Acetyl-alpha-D-glucosamine-enolpyruvate is reduced through a NADPH dependent UDP-N-acetylenolpyruvoylglucosamine reductase, releasing a NADP and a UDP-N-acetyl-alpha-D-muramate. This compound is involved in the D-glutamine and D-glutamate metabolism.
References
Amino Sugar and Nucleotide Sugar Metabolism III References
Souza JM, Plumbridge JA, Calcagno ML: N-acetylglucosamine-6-phosphate deacetylase from Escherichia coli: purification and molecular and kinetic characterization. Arch Biochem Biophys. 1997 Apr 15;340(2):338-46. doi: 10.1006/abbi.1997.9780.
Pubmed: 9143339
Ferreira FM, Mendoza-Hernandez G, Castaneda-Bueno M, Aparicio R, Fischer H, Calcagno ML, Oliva G: Structural analysis of N-acetylglucosamine-6-phosphate deacetylase apoenzyme from Escherichia coli. J Mol Biol. 2006 Jun 2;359(2):308-21. doi: 10.1016/j.jmb.2006.03.024. Epub 2006 Mar 29.
Pubmed: 16630633
Plumbridge JA: Sequence of the nagBACD operon in Escherichia coli K12 and pattern of transcription within the nag regulon. Mol Microbiol. 1989 Apr;3(4):505-15. doi: 10.1111/j.1365-2958.1989.tb00197.x.
Pubmed: 2668691
Rogers MJ, Ohgi T, Plumbridge J, Soll D: Nucleotide sequences of the Escherichia coli nagE and nagB genes: the structural genes for the N-acetylglucosamine transport protein of the bacterial phosphoenolpyruvate: sugar phosphotransferase system and for glucosamine-6-phosphate deaminase. Gene. 1988;62(2):197-207. doi: 10.1016/0378-1119(88)90558-6.
Pubmed: 3284790
Altamirano MM, Plumbridge JA, Barba HA, Calcagno ML: Glucosamine-6-phosphate deaminase from Escherichia coli has a trimer of dimers structure with three intersubunit disulphides. Biochem J. 1993 Nov 1;295 ( Pt 3):645-8. doi: 10.1042/bj2950645.
Pubmed: 8240271
Peri KG, Goldie H, Waygood EB: Cloning and characterization of the N-acetylglucosamine operon of Escherichia coli. Biochem Cell Biol. 1990 Jan;68(1):123-37.
Pubmed: 2190615
Uehara T, Park JT: The N-acetyl-D-glucosamine kinase of Escherichia coli and its role in murein recycling. J Bacteriol. 2004 Nov;186(21):7273-9. doi: 10.1128/JB.186.21.7273-7279.2004.
Pubmed: 15489439
Oshima T, Aiba H, Baba T, Fujita K, Hayashi K, Honjo A, Ikemoto K, Inada T, Itoh T, Kajihara M, Kanai K, Kashimoto K, Kimura S, Kitagawa M, Makino K, Masuda S, Miki T, Mizobuchi K, Mori H, Motomura K, Nakamura Y, Nashimoto H, Nishio Y, Saito N, Horiuchi T, et al.: A 718-kb DNA sequence of the Escherichia coli K-12 genome corresponding to the 12.7-28.0 min region on the linkage map. DNA Res. 1996 Jun 30;3(3):137-55. doi: 10.1093/dnares/3.3.137.
Pubmed: 8905232
Blattner FR, Plunkett G 3rd, Bloch CA, Perna NT, Burland V, Riley M, Collado-Vides J, Glasner JD, Rode CK, Mayhew GF, Gregor J, Davis NW, Kirkpatrick HA, Goeden MA, Rose DJ, Mau B, Shao Y: The complete genome sequence of Escherichia coli K-12. Science. 1997 Sep 5;277(5331):1453-62. doi: 10.1126/science.277.5331.1453.
Pubmed: 9278503
Hayashi K, Morooka N, Yamamoto Y, Fujita K, Isono K, Choi S, Ohtsubo E, Baba T, Wanner BL, Mori H, Horiuchi T: Highly accurate genome sequences of Escherichia coli K-12 strains MG1655 and W3110. Mol Syst Biol. 2006;2:2006.0007. doi: 10.1038/msb4100049. Epub 2006 Feb 21.
Pubmed: 16738553
Andrews SC, Harrison PM, Guest JR: Cloning, sequencing, and mapping of the bacterioferritin gene (bfr) of Escherichia coli K-12. J Bacteriol. 1989 Jul;171(7):3940-7. doi: 10.1128/jb.171.7.3940-3947.1989.
Pubmed: 2661540
Wong LJ, Sheu KF, Lee SL, Frey PA: Galactose-1-phosphate uridylyltransferase: isolation and properties of a uridylyl-enzyme intermediate. Biochemistry. 1977 Mar 8;16(5):1010-6. doi: 10.1021/bi00624a032.
Pubmed: 321007
Lemaire HG, Muller-Hill B: Nucleotide sequences of the gal E gene and the gal T gene of E. coli. Nucleic Acids Res. 1986 Oct 10;14(19):7705-11. doi: 10.1093/nar/14.19.7705.
Pubmed: 3022232
Aiba H, Baba T, Hayashi K, Inada T, Isono K, Itoh T, Kasai H, Kashimoto K, Kimura S, Kitakawa M, Kitagawa M, Makino K, Miki T, Mizobuchi K, Mori H, Mori T, Motomura K, Nakade S, Nakamura Y, Nashimoto H, Nishio Y, Oshima T, Saito N, Sampei G, Horiuchi T, et al.: A 570-kb DNA sequence of the Escherichia coli K-12 genome corresponding to the 28.0-40.1 min region on the linkage map. DNA Res. 1996 Dec 31;3(6):363-77. doi: 10.1093/dnares/3.6.363.
Pubmed: 9097039
Ueguchi C, Ito K: Multicopy suppression: an approach to understanding intracellular functioning of the protein export system. J Bacteriol. 1992 Mar;174(5):1454-61. doi: 10.1128/jb.174.5.1454-1461.1992.
Pubmed: 1537791
Weissborn AC, Liu Q, Rumley MK, Kennedy EP: UTP: alpha-D-glucose-1-phosphate uridylyltransferase of Escherichia coli: isolation and DNA sequence of the galU gene and purification of the enzyme. J Bacteriol. 1994 May;176(9):2611-8. doi: 10.1128/jb.176.9.2611-2618.1994.
Pubmed: 8169209
Hossain SA, Tanizawa K, Kazuta Y, Fukui T: Overproduction and characterization of recombinant UDP-glucose pyrophosphorylase from Escherichia coli K-12. J Biochem. 1994 May;115(5):965-72. doi: 10.1093/oxfordjournals.jbchem.a124446.
Pubmed: 7961613
Itoh T, Aiba H, Baba T, Hayashi K, Inada T, Isono K, Kasai H, Kimura S, Kitakawa M, Kitagawa M, Makino K, Miki T, Mizobuchi K, Mori H, Mori T, Motomura K, Nakade S, Nakamura Y, Nashimoto H, Nishio Y, Oshima T, Saito N, Sampei G, Seki Y, Horiuchi T, et al.: A 460-kb DNA sequence of the Escherichia coli K-12 genome corresponding to the 40.1-50.0 min region on the linkage map. DNA Res. 1996 Dec 31;3(6):379-92. doi: 10.1093/dnares/3.6.379.
Pubmed: 9097040
This pathway was propagated using PathWhiz -
Pon, A. et al. Pathways with PathWhiz (2015) Nucleic Acids Res. 43(Web Server issue): W552–W559.
Propagated from SMP0000914
Highlighted elements will appear in red.
Highlight Compounds
Highlight Proteins
Enter relative concentration values (without units). Elements will be highlighted in a color gradient where red = lowest concentration and green = highest concentration. For the best results, view the pathway in Black and White.
Visualize Compound Data
Visualize Protein Data
Settings