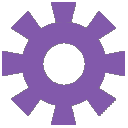
Browsing Pathways
Showing 326071 -
326080 of 605359 pathways
PathBank ID | Pathway Name and Description | Pathway Class | Chemical Compounds | Proteins |
---|---|---|---|---|
SMP0357096![]() |
Cardiolipin Biosynthesis CL(i-12:0/a-13:0/i-15:0/i-24:0)[rac]Homo sapiens
Cardiolipin (CL) is an important component of the inner mitochondrial membrane where it constitutes about 20% of the total lipid composition. It is essential for the optimal function of numerous enzymes that are involved in mitochondrial energy metabolism (Wikipedia). Cardiolipin biosynthesis occurs mainly in the mitochondria, but there also exists an alternative synthesis route for CDP-diacylglycerol that takes place in the endoplasmic reticulum. This second route may supplement this pathway. All membrane-localized enzymes are coloured dark green in the image. First, dihydroxyacetone phosphate (or glycerone phosphate) from glycolysis is used by the cytosolic enzyme glycerol-3-phosphate dehydrogenase [NAD(+)] to synthesize sn-glycerol 3-phosphate. Second, the mitochondrial outer membrane enzyme glycerol-3-phosphate acyltransferase esterifies an acyl-group to the sn-1 position of sn-glycerol 3-phosphate to form 1-acyl-sn-glycerol 3-phosphate (lysophosphatidic acid or LPA). Third, the enzyme 1-acyl-sn-glycerol-3-phosphate acyltransferase converts LPA into phosphatidic acid (PA or 1,2-diacyl-sn-glycerol 3-phosphate) by esterifying an acyl-group to the sn-2 position of the glycerol backbone. PA is then transferred to the inner mitochondrial membrane to continue cardiolipin synthesis. Fourth, magnesium-dependent phosphatidate cytidylyltransferase catalyzes the conversion of PA into CDP-diacylglycerol. Fifth, CDP-diacylglycerol--glycerol-3-phosphate 3-phosphatidyltransferase synthesizes phosphatidylglycerophosphate (PGP). Sixth, phosphatidylglycerophosphatase and protein-tyrosine phosphatase dephosphorylates PGP to form phosphatidylglycerol (PG). Last, cardiolipin synthase catalyzes the synthesis of cardiolipin by transferring a phosphatidyl group from a second CDP-diacylglycerol to PG. It requires a divalent metal cation cofactor.
|
Metabolite
Metabolic
|
|
|
SMP0357089![]() |
Cardiolipin Biosynthesis CL(i-12:0/a-13:0/i-15:0/i-19:0)[rac]Homo sapiens
Cardiolipin (CL) is an important component of the inner mitochondrial membrane where it constitutes about 20% of the total lipid composition. It is essential for the optimal function of numerous enzymes that are involved in mitochondrial energy metabolism (Wikipedia). Cardiolipin biosynthesis occurs mainly in the mitochondria, but there also exists an alternative synthesis route for CDP-diacylglycerol that takes place in the endoplasmic reticulum. This second route may supplement this pathway. All membrane-localized enzymes are coloured dark green in the image. First, dihydroxyacetone phosphate (or glycerone phosphate) from glycolysis is used by the cytosolic enzyme glycerol-3-phosphate dehydrogenase [NAD(+)] to synthesize sn-glycerol 3-phosphate. Second, the mitochondrial outer membrane enzyme glycerol-3-phosphate acyltransferase esterifies an acyl-group to the sn-1 position of sn-glycerol 3-phosphate to form 1-acyl-sn-glycerol 3-phosphate (lysophosphatidic acid or LPA). Third, the enzyme 1-acyl-sn-glycerol-3-phosphate acyltransferase converts LPA into phosphatidic acid (PA or 1,2-diacyl-sn-glycerol 3-phosphate) by esterifying an acyl-group to the sn-2 position of the glycerol backbone. PA is then transferred to the inner mitochondrial membrane to continue cardiolipin synthesis. Fourth, magnesium-dependent phosphatidate cytidylyltransferase catalyzes the conversion of PA into CDP-diacylglycerol. Fifth, CDP-diacylglycerol--glycerol-3-phosphate 3-phosphatidyltransferase synthesizes phosphatidylglycerophosphate (PGP). Sixth, phosphatidylglycerophosphatase and protein-tyrosine phosphatase dephosphorylates PGP to form phosphatidylglycerol (PG). Last, cardiolipin synthase catalyzes the synthesis of cardiolipin by transferring a phosphatidyl group from a second CDP-diacylglycerol to PG. It requires a divalent metal cation cofactor.
|
Metabolite
Metabolic
|
|
|
SMP0357116![]() |
Cardiolipin Biosynthesis CL(i-12:0/a-13:0/i-16:0/i-14:0)[rac]Homo sapiens
Cardiolipin (CL) is an important component of the inner mitochondrial membrane where it constitutes about 20% of the total lipid composition. It is essential for the optimal function of numerous enzymes that are involved in mitochondrial energy metabolism (Wikipedia). Cardiolipin biosynthesis occurs mainly in the mitochondria, but there also exists an alternative synthesis route for CDP-diacylglycerol that takes place in the endoplasmic reticulum. This second route may supplement this pathway. All membrane-localized enzymes are coloured dark green in the image. First, dihydroxyacetone phosphate (or glycerone phosphate) from glycolysis is used by the cytosolic enzyme glycerol-3-phosphate dehydrogenase [NAD(+)] to synthesize sn-glycerol 3-phosphate. Second, the mitochondrial outer membrane enzyme glycerol-3-phosphate acyltransferase esterifies an acyl-group to the sn-1 position of sn-glycerol 3-phosphate to form 1-acyl-sn-glycerol 3-phosphate (lysophosphatidic acid or LPA). Third, the enzyme 1-acyl-sn-glycerol-3-phosphate acyltransferase converts LPA into phosphatidic acid (PA or 1,2-diacyl-sn-glycerol 3-phosphate) by esterifying an acyl-group to the sn-2 position of the glycerol backbone. PA is then transferred to the inner mitochondrial membrane to continue cardiolipin synthesis. Fourth, magnesium-dependent phosphatidate cytidylyltransferase catalyzes the conversion of PA into CDP-diacylglycerol. Fifth, CDP-diacylglycerol--glycerol-3-phosphate 3-phosphatidyltransferase synthesizes phosphatidylglycerophosphate (PGP). Sixth, phosphatidylglycerophosphatase and protein-tyrosine phosphatase dephosphorylates PGP to form phosphatidylglycerol (PG). Last, cardiolipin synthase catalyzes the synthesis of cardiolipin by transferring a phosphatidyl group from a second CDP-diacylglycerol to PG. It requires a divalent metal cation cofactor.
|
Metabolite
Metabolic
|
|
|
SMP0357091![]() |
Cardiolipin Biosynthesis CL(i-12:0/a-13:0/i-15:0/i-20:0)[rac]Homo sapiens
Cardiolipin (CL) is an important component of the inner mitochondrial membrane where it constitutes about 20% of the total lipid composition. It is essential for the optimal function of numerous enzymes that are involved in mitochondrial energy metabolism (Wikipedia). Cardiolipin biosynthesis occurs mainly in the mitochondria, but there also exists an alternative synthesis route for CDP-diacylglycerol that takes place in the endoplasmic reticulum. This second route may supplement this pathway. All membrane-localized enzymes are coloured dark green in the image. First, dihydroxyacetone phosphate (or glycerone phosphate) from glycolysis is used by the cytosolic enzyme glycerol-3-phosphate dehydrogenase [NAD(+)] to synthesize sn-glycerol 3-phosphate. Second, the mitochondrial outer membrane enzyme glycerol-3-phosphate acyltransferase esterifies an acyl-group to the sn-1 position of sn-glycerol 3-phosphate to form 1-acyl-sn-glycerol 3-phosphate (lysophosphatidic acid or LPA). Third, the enzyme 1-acyl-sn-glycerol-3-phosphate acyltransferase converts LPA into phosphatidic acid (PA or 1,2-diacyl-sn-glycerol 3-phosphate) by esterifying an acyl-group to the sn-2 position of the glycerol backbone. PA is then transferred to the inner mitochondrial membrane to continue cardiolipin synthesis. Fourth, magnesium-dependent phosphatidate cytidylyltransferase catalyzes the conversion of PA into CDP-diacylglycerol. Fifth, CDP-diacylglycerol--glycerol-3-phosphate 3-phosphatidyltransferase synthesizes phosphatidylglycerophosphate (PGP). Sixth, phosphatidylglycerophosphatase and protein-tyrosine phosphatase dephosphorylates PGP to form phosphatidylglycerol (PG). Last, cardiolipin synthase catalyzes the synthesis of cardiolipin by transferring a phosphatidyl group from a second CDP-diacylglycerol to PG. It requires a divalent metal cation cofactor.
|
Metabolite
Metabolic
|
|
|
SMP0356751![]() |
Ethylene Glycol DegradationPrevotella nigrescens ATCC 33563
Ethylene glycol, or 1,2-ethanediol, is used to produce substances such as plastics, solvents, surfactants, explosives and cosmetics. Many of these are discarded into waste treatment and landfills. Both aerobic and anaerobic microorganisms can degrade ethylene glycol. While ethylene glycol cannot be used as a carbon source by wild-type E.coli, it can be utilized by isolated mutant strains. These strains contain two regulatory mutations: a mutation that increases propanediol oxidoreductase levels which functions to metabolize propanediol, and increased activity of Glycolaldehyde dehydrogenase to produce glycolate from glycolaldehyde.
|
Metabolite
Metabolic
|
||
SMP0356744![]() |
Ethylene Glycol DegradationPrevotella disiens FB035-09AN
Ethylene glycol, or 1,2-ethanediol, is used to produce substances such as plastics, solvents, surfactants, explosives and cosmetics. Many of these are discarded into waste treatment and landfills. Both aerobic and anaerobic microorganisms can degrade ethylene glycol. While ethylene glycol cannot be used as a carbon source by wild-type E.coli, it can be utilized by isolated mutant strains. These strains contain two regulatory mutations: a mutation that increases propanediol oxidoreductase levels which functions to metabolize propanediol, and increased activity of Glycolaldehyde dehydrogenase to produce glycolate from glycolaldehyde.
|
Metabolite
Metabolic
|
||
SMP0356693![]() |
Ethylene Glycol DegradationBacteroides caccae ATCC 43185
Ethylene glycol, or 1,2-ethanediol, is used to produce substances such as plastics, solvents, surfactants, explosives and cosmetics. Many of these are discarded into waste treatment and landfills. Both aerobic and anaerobic microorganisms can degrade ethylene glycol. While ethylene glycol cannot be used as a carbon source by wild-type E.coli, it can be utilized by isolated mutant strains. These strains contain two regulatory mutations: a mutation that increases propanediol oxidoreductase levels which functions to metabolize propanediol, and increased activity of Glycolaldehyde dehydrogenase to produce glycolate from glycolaldehyde.
|
Metabolite
Metabolic
|
||
SMP0356698![]() |
Ethylene Glycol DegradationBacteroides ovatus ATCC 8483
Ethylene glycol, or 1,2-ethanediol, is used to produce substances such as plastics, solvents, surfactants, explosives and cosmetics. Many of these are discarded into waste treatment and landfills. Both aerobic and anaerobic microorganisms can degrade ethylene glycol. While ethylene glycol cannot be used as a carbon source by wild-type E.coli, it can be utilized by isolated mutant strains. These strains contain two regulatory mutations: a mutation that increases propanediol oxidoreductase levels which functions to metabolize propanediol, and increased activity of Glycolaldehyde dehydrogenase to produce glycolate from glycolaldehyde.
|
Metabolite
Metabolic
|
||
SMP0349798 |
Ethylene Glycol DegradationTannerella forsythia
Ethylene glycol, or 1,2-ethanediol, is used to produce substances such as plastics, solvents, surfactants, explosives and cosmetics. Many of these are discarded into waste treatment and landfills. Both aerobic and anaerobic microorganisms can degrade ethylene glycol. While ethylene glycol cannot be used as a carbon source by wild-type E.coli, it can be utilized by isolated mutant strains. These strains contain two regulatory mutations: a mutation that increases propanediol oxidoreductase levels which functions to metabolize propanediol, and increased activity of Glycolaldehyde dehydrogenase to produce glycolate from glycolaldehyde.
|
Metabolite
Metabolic
|
||
SMP0356767![]() |
NAD Phosphorylation and DephosphorylationBuchnera aphidicola str. APS (Acyrthosiphon pisum)
NAD kinase is required for converting NAD to NADP in various organisms such as groups of archaea, eubacteria and eukaryotes. For example, NAD kinase has shown its important role for the growth in Salmonella enterica and the importance in E.coli. NADP can be converted back to NAD via facilitation of alkaline phosphatase with water (hydroxylation).
|
Metabolite
Metabolic
|
Showing 326071 -
326080 of 326198 pathways