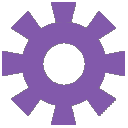
Browsing Pathways
Showing 346061 -
346070 of 605359 pathways
PathBank ID | Pathway Name and Description | Pathway Class | Chemical Compounds | Proteins |
---|---|---|---|---|
SMP0214787 |
Cardiolipin Biosynthesis CL(18:1(9Z)/18:2(9Z,12Z)/16:0/18:4(6Z,9Z,12Z,15Z))Homo sapiens
Cardiolipin (CL) is an important component of the inner mitochondrial membrane where it constitutes about 20% of the total lipid composition. It is essential for the optimal function of numerous enzymes that are involved in mitochondrial energy metabolism (Wikipedia). Cardiolipin biosynthesis occurs mainly in the mitochondria, but there also exists an alternative synthesis route for CDP-diacylglycerol that takes place in the endoplasmic reticulum. This second route may supplement this pathway. All membrane-localized enzymes are coloured dark green in the image. First, dihydroxyacetone phosphate (or glycerone phosphate) from glycolysis is used by the cytosolic enzyme glycerol-3-phosphate dehydrogenase [NAD(+)] to synthesize sn-glycerol 3-phosphate. Second, the mitochondrial outer membrane enzyme glycerol-3-phosphate acyltransferase esterifies an acyl-group to the sn-1 position of sn-glycerol 3-phosphate to form 1-acyl-sn-glycerol 3-phosphate (lysophosphatidic acid or LPA). Third, the enzyme 1-acyl-sn-glycerol-3-phosphate acyltransferase converts LPA into phosphatidic acid (PA or 1,2-diacyl-sn-glycerol 3-phosphate) by esterifying an acyl-group to the sn-2 position of the glycerol backbone. PA is then transferred to the inner mitochondrial membrane to continue cardiolipin synthesis. Fourth, magnesium-dependent phosphatidate cytidylyltransferase catalyzes the conversion of PA into CDP-diacylglycerol. Fifth, CDP-diacylglycerol--glycerol-3-phosphate 3-phosphatidyltransferase synthesizes phosphatidylglycerophosphate (PGP). Sixth, phosphatidylglycerophosphatase and protein-tyrosine phosphatase dephosphorylates PGP to form phosphatidylglycerol (PG). Last, cardiolipin synthase catalyzes the synthesis of cardiolipin by transferring a phosphatidyl group from a second CDP-diacylglycerol to PG. It requires a divalent metal cation cofactor.
|
Metabolite
Metabolic
|
|
|
SMP0125531 |
Bile Acid Biosynthesis 1662027393Homo sapiens
A bile acids life begins as cholesterol is catabolized, as bile acid is a derivative of cholesterol. This pathway occurs in the liver, beginning with cholesterol being converted to 7a-hydroxycholesterol through the enzyme cholesterol-7-alpha-monooxygenase, after being transported into the liver cell. 7a-hydroxycholesterol then becomes 7a-hydroxy-cholestene-3-one, which is made possible by the enzyme 3-beta-hydroxysteroid dehydrogenase type 7. 7a-hydroxy-cholestene-3-one then is used in two different chains of reactions. The first, continuing in the liver, uses the enzyme 3-oxo-5-beta-steroid-4-deydrogenase to become 7a-hydroxy-5b-cholestan-3-one. After that, aldo-keto reductase family 1 member C4 is used to create 3a,7a-dihydroxy-5b-cholestane. In the mitochondria of the cell, sterol 26-hydroxylase converts 3a,7a-dihydroxy-5b-cholestane to 3a,7a,26-trihydroxy-5b-cholestane, which is then converted to 3a,7a-dihydroxy-5b-cholestan-26-al by the same enzyme used in the previous reaction. This enzyme is used another time, to create 3a,7a-dihydroxycoprostanic acid. Then, bile acyl-CoA synthetase teams up with 3a,7a-dihydroxycoprostanic acid to create 3a,7a-dihydroxy-5b-cholestanoyl-CoA. 3a,7a-dihydroxy-5b-cholestanoyl-CoA remains intact while alpha-methylacyl-CoA racemase moves it along through the peroxisome. Peroxisomal acyl coenzyme A oxidase 2 converts 3a,7a-dihydroxy-5b-cholestanoyl-CoA into 3a,7a-dihydoxy-5b-cholest-24-enoyl-CoA. With the help of water, peroxisomal multifunctional enzyme type 2 turns 3a,7a-dihydoxy-5b-cholest-24-enoyl-CoA into 3a,7a,24-trihydoxy-5b-cholestanoyl-CoA. This compound then uses peroxisomal multifunctional enzyme type 2 to create chenodeoxycholoyl-CoA. From there, propionyl-CoA and chenodeoxycholoyl-CoA join forces and enlist the help of non-specific lipid transfer protein to further chenodeoxycholoyl-CoAâ€TMs journey in the peroxisome. It is then transported back into intracellular space, where after its used in 3 different reactions, its derivatives interact with intestinal microflora in the extracellular space to become lithocholyltaurine, lithocholic acid glycine conjugate, and lithocholic acid. Revisiting 7a-hydroxy-cholestene-3-one, the second chain of reactions it is involved in follows a similar path as the first, moving through the mitochondria, endoplasmic reticulum and peroxisome until choloyl-CoA is formed, which then is used in three reactions so that its derivatives may leave the cell to interact with intestinal microflora and become taurodeoxycholic acid, deoxycholic acid glycine conjugate and deoxycholic acid. There are two more important components of this pathway, both depicting the breakdown of cholesterol into bile acid. These components of the pathway occur in the endoplasmic reticulum membrane, although 2 enzymes, 25-hydroxycholesterol 7-alpha-hydroxylase and sterol 26 hydroxylase, are found in the mitochondria. Bile acids play a very important part in the digestion of foods, and are responsible for the absorption of water soluble vitamins in the small intestine. Bile acids also help absorb fats into the small intestine, a crucial part of any vertebrates diet.
|
Metabolite
Metabolic
|
|
|
SMP0218786![]() |
Cardiolipin Biosynthesis CL(18:2(9Z,12Z)/22:5(7Z,10Z,13Z,16Z,19Z)/20:4(5Z,8Z,11Z,14Z)/16:0)Homo sapiens
Cardiolipin (CL) is an important component of the inner mitochondrial membrane where it constitutes about 20% of the total lipid composition. It is essential for the optimal function of numerous enzymes that are involved in mitochondrial energy metabolism (Wikipedia). Cardiolipin biosynthesis occurs mainly in the mitochondria, but there also exists an alternative synthesis route for CDP-diacylglycerol that takes place in the endoplasmic reticulum. This second route may supplement this pathway. All membrane-localized enzymes are coloured dark green in the image. First, dihydroxyacetone phosphate (or glycerone phosphate) from glycolysis is used by the cytosolic enzyme glycerol-3-phosphate dehydrogenase [NAD(+)] to synthesize sn-glycerol 3-phosphate. Second, the mitochondrial outer membrane enzyme glycerol-3-phosphate acyltransferase esterifies an acyl-group to the sn-1 position of sn-glycerol 3-phosphate to form 1-acyl-sn-glycerol 3-phosphate (lysophosphatidic acid or LPA). Third, the enzyme 1-acyl-sn-glycerol-3-phosphate acyltransferase converts LPA into phosphatidic acid (PA or 1,2-diacyl-sn-glycerol 3-phosphate) by esterifying an acyl-group to the sn-2 position of the glycerol backbone. PA is then transferred to the inner mitochondrial membrane to continue cardiolipin synthesis. Fourth, magnesium-dependent phosphatidate cytidylyltransferase catalyzes the conversion of PA into CDP-diacylglycerol. Fifth, CDP-diacylglycerol--glycerol-3-phosphate 3-phosphatidyltransferase synthesizes phosphatidylglycerophosphate (PGP). Sixth, phosphatidylglycerophosphatase and protein-tyrosine phosphatase dephosphorylates PGP to form phosphatidylglycerol (PG). Last, cardiolipin synthase catalyzes the synthesis of cardiolipin by transferring a phosphatidyl group from a second CDP-diacylglycerol to PG. It requires a divalent metal cation cofactor.
|
Metabolite
Metabolic
|
|
|
SMP0218788![]() |
Cardiolipin Biosynthesis CL(18:2(9Z,12Z)/22:5(7Z,10Z,13Z,16Z,19Z)/20:4(5Z,8Z,11Z,14Z)/18:0)Homo sapiens
Cardiolipin (CL) is an important component of the inner mitochondrial membrane where it constitutes about 20% of the total lipid composition. It is essential for the optimal function of numerous enzymes that are involved in mitochondrial energy metabolism (Wikipedia). Cardiolipin biosynthesis occurs mainly in the mitochondria, but there also exists an alternative synthesis route for CDP-diacylglycerol that takes place in the endoplasmic reticulum. This second route may supplement this pathway. All membrane-localized enzymes are coloured dark green in the image. First, dihydroxyacetone phosphate (or glycerone phosphate) from glycolysis is used by the cytosolic enzyme glycerol-3-phosphate dehydrogenase [NAD(+)] to synthesize sn-glycerol 3-phosphate. Second, the mitochondrial outer membrane enzyme glycerol-3-phosphate acyltransferase esterifies an acyl-group to the sn-1 position of sn-glycerol 3-phosphate to form 1-acyl-sn-glycerol 3-phosphate (lysophosphatidic acid or LPA). Third, the enzyme 1-acyl-sn-glycerol-3-phosphate acyltransferase converts LPA into phosphatidic acid (PA or 1,2-diacyl-sn-glycerol 3-phosphate) by esterifying an acyl-group to the sn-2 position of the glycerol backbone. PA is then transferred to the inner mitochondrial membrane to continue cardiolipin synthesis. Fourth, magnesium-dependent phosphatidate cytidylyltransferase catalyzes the conversion of PA into CDP-diacylglycerol. Fifth, CDP-diacylglycerol--glycerol-3-phosphate 3-phosphatidyltransferase synthesizes phosphatidylglycerophosphate (PGP). Sixth, phosphatidylglycerophosphatase and protein-tyrosine phosphatase dephosphorylates PGP to form phosphatidylglycerol (PG). Last, cardiolipin synthase catalyzes the synthesis of cardiolipin by transferring a phosphatidyl group from a second CDP-diacylglycerol to PG. It requires a divalent metal cation cofactor.
|
Metabolite
Metabolic
|
|
|
SMP0218793![]() |
Cardiolipin Biosynthesis CL(18:2(9Z,12Z)/22:5(7Z,10Z,13Z,16Z,19Z)/20:4(5Z,8Z,11Z,14Z)/20:5(5Z,8Z,11Z,14Z,17Z))Homo sapiens
Cardiolipin (CL) is an important component of the inner mitochondrial membrane where it constitutes about 20% of the total lipid composition. It is essential for the optimal function of numerous enzymes that are involved in mitochondrial energy metabolism (Wikipedia). Cardiolipin biosynthesis occurs mainly in the mitochondria, but there also exists an alternative synthesis route for CDP-diacylglycerol that takes place in the endoplasmic reticulum. This second route may supplement this pathway. All membrane-localized enzymes are coloured dark green in the image. First, dihydroxyacetone phosphate (or glycerone phosphate) from glycolysis is used by the cytosolic enzyme glycerol-3-phosphate dehydrogenase [NAD(+)] to synthesize sn-glycerol 3-phosphate. Second, the mitochondrial outer membrane enzyme glycerol-3-phosphate acyltransferase esterifies an acyl-group to the sn-1 position of sn-glycerol 3-phosphate to form 1-acyl-sn-glycerol 3-phosphate (lysophosphatidic acid or LPA). Third, the enzyme 1-acyl-sn-glycerol-3-phosphate acyltransferase converts LPA into phosphatidic acid (PA or 1,2-diacyl-sn-glycerol 3-phosphate) by esterifying an acyl-group to the sn-2 position of the glycerol backbone. PA is then transferred to the inner mitochondrial membrane to continue cardiolipin synthesis. Fourth, magnesium-dependent phosphatidate cytidylyltransferase catalyzes the conversion of PA into CDP-diacylglycerol. Fifth, CDP-diacylglycerol--glycerol-3-phosphate 3-phosphatidyltransferase synthesizes phosphatidylglycerophosphate (PGP). Sixth, phosphatidylglycerophosphatase and protein-tyrosine phosphatase dephosphorylates PGP to form phosphatidylglycerol (PG). Last, cardiolipin synthase catalyzes the synthesis of cardiolipin by transferring a phosphatidyl group from a second CDP-diacylglycerol to PG. It requires a divalent metal cation cofactor.
|
Metabolite
Metabolic
|
|
|
SMP0218685![]() |
Cardiolipin Biosynthesis CL(18:2(9Z,12Z)/22:5(7Z,10Z,13Z,16Z,19Z)/16:0/20:5(5Z,8Z,11Z,14Z,17Z))Homo sapiens
Cardiolipin (CL) is an important component of the inner mitochondrial membrane where it constitutes about 20% of the total lipid composition. It is essential for the optimal function of numerous enzymes that are involved in mitochondrial energy metabolism (Wikipedia). Cardiolipin biosynthesis occurs mainly in the mitochondria, but there also exists an alternative synthesis route for CDP-diacylglycerol that takes place in the endoplasmic reticulum. This second route may supplement this pathway. All membrane-localized enzymes are coloured dark green in the image. First, dihydroxyacetone phosphate (or glycerone phosphate) from glycolysis is used by the cytosolic enzyme glycerol-3-phosphate dehydrogenase [NAD(+)] to synthesize sn-glycerol 3-phosphate. Second, the mitochondrial outer membrane enzyme glycerol-3-phosphate acyltransferase esterifies an acyl-group to the sn-1 position of sn-glycerol 3-phosphate to form 1-acyl-sn-glycerol 3-phosphate (lysophosphatidic acid or LPA). Third, the enzyme 1-acyl-sn-glycerol-3-phosphate acyltransferase converts LPA into phosphatidic acid (PA or 1,2-diacyl-sn-glycerol 3-phosphate) by esterifying an acyl-group to the sn-2 position of the glycerol backbone. PA is then transferred to the inner mitochondrial membrane to continue cardiolipin synthesis. Fourth, magnesium-dependent phosphatidate cytidylyltransferase catalyzes the conversion of PA into CDP-diacylglycerol. Fifth, CDP-diacylglycerol--glycerol-3-phosphate 3-phosphatidyltransferase synthesizes phosphatidylglycerophosphate (PGP). Sixth, phosphatidylglycerophosphatase and protein-tyrosine phosphatase dephosphorylates PGP to form phosphatidylglycerol (PG). Last, cardiolipin synthase catalyzes the synthesis of cardiolipin by transferring a phosphatidyl group from a second CDP-diacylglycerol to PG. It requires a divalent metal cation cofactor.
|
Metabolite
Metabolic
|
|
|
SMP0218687![]() |
Cardiolipin Biosynthesis CL(18:2(9Z,12Z)/22:5(7Z,10Z,13Z,16Z,19Z)/16:0/22:5(7Z,10Z,13Z,16Z,19Z))Homo sapiens
Cardiolipin (CL) is an important component of the inner mitochondrial membrane where it constitutes about 20% of the total lipid composition. It is essential for the optimal function of numerous enzymes that are involved in mitochondrial energy metabolism (Wikipedia). Cardiolipin biosynthesis occurs mainly in the mitochondria, but there also exists an alternative synthesis route for CDP-diacylglycerol that takes place in the endoplasmic reticulum. This second route may supplement this pathway. All membrane-localized enzymes are coloured dark green in the image. First, dihydroxyacetone phosphate (or glycerone phosphate) from glycolysis is used by the cytosolic enzyme glycerol-3-phosphate dehydrogenase [NAD(+)] to synthesize sn-glycerol 3-phosphate. Second, the mitochondrial outer membrane enzyme glycerol-3-phosphate acyltransferase esterifies an acyl-group to the sn-1 position of sn-glycerol 3-phosphate to form 1-acyl-sn-glycerol 3-phosphate (lysophosphatidic acid or LPA). Third, the enzyme 1-acyl-sn-glycerol-3-phosphate acyltransferase converts LPA into phosphatidic acid (PA or 1,2-diacyl-sn-glycerol 3-phosphate) by esterifying an acyl-group to the sn-2 position of the glycerol backbone. PA is then transferred to the inner mitochondrial membrane to continue cardiolipin synthesis. Fourth, magnesium-dependent phosphatidate cytidylyltransferase catalyzes the conversion of PA into CDP-diacylglycerol. Fifth, CDP-diacylglycerol--glycerol-3-phosphate 3-phosphatidyltransferase synthesizes phosphatidylglycerophosphate (PGP). Sixth, phosphatidylglycerophosphatase and protein-tyrosine phosphatase dephosphorylates PGP to form phosphatidylglycerol (PG). Last, cardiolipin synthase catalyzes the synthesis of cardiolipin by transferring a phosphatidyl group from a second CDP-diacylglycerol to PG. It requires a divalent metal cation cofactor.
|
Metabolite
Metabolic
|
|
|
SMP0218692![]() |
Cardiolipin Biosynthesis CL(18:2(9Z,12Z)/22:5(7Z,10Z,13Z,16Z,19Z)/16:1(9Z)/18:0)Homo sapiens
Cardiolipin (CL) is an important component of the inner mitochondrial membrane where it constitutes about 20% of the total lipid composition. It is essential for the optimal function of numerous enzymes that are involved in mitochondrial energy metabolism (Wikipedia). Cardiolipin biosynthesis occurs mainly in the mitochondria, but there also exists an alternative synthesis route for CDP-diacylglycerol that takes place in the endoplasmic reticulum. This second route may supplement this pathway. All membrane-localized enzymes are coloured dark green in the image. First, dihydroxyacetone phosphate (or glycerone phosphate) from glycolysis is used by the cytosolic enzyme glycerol-3-phosphate dehydrogenase [NAD(+)] to synthesize sn-glycerol 3-phosphate. Second, the mitochondrial outer membrane enzyme glycerol-3-phosphate acyltransferase esterifies an acyl-group to the sn-1 position of sn-glycerol 3-phosphate to form 1-acyl-sn-glycerol 3-phosphate (lysophosphatidic acid or LPA). Third, the enzyme 1-acyl-sn-glycerol-3-phosphate acyltransferase converts LPA into phosphatidic acid (PA or 1,2-diacyl-sn-glycerol 3-phosphate) by esterifying an acyl-group to the sn-2 position of the glycerol backbone. PA is then transferred to the inner mitochondrial membrane to continue cardiolipin synthesis. Fourth, magnesium-dependent phosphatidate cytidylyltransferase catalyzes the conversion of PA into CDP-diacylglycerol. Fifth, CDP-diacylglycerol--glycerol-3-phosphate 3-phosphatidyltransferase synthesizes phosphatidylglycerophosphate (PGP). Sixth, phosphatidylglycerophosphatase and protein-tyrosine phosphatase dephosphorylates PGP to form phosphatidylglycerol (PG). Last, cardiolipin synthase catalyzes the synthesis of cardiolipin by transferring a phosphatidyl group from a second CDP-diacylglycerol to PG. It requires a divalent metal cation cofactor.
|
Metabolite
Metabolic
|
|
|
SMP0126624 |
Citric Acid Cycle 1691135724Caenorhabditis elegans
The citric acid cycle, which is also known as the tricarboxylic acid cycle (TCA cycle) or the Krebs cycle, is a connected series of enzyme-catalyzed chemical reactions of central importance to all aerobic organisms (i.e. organisms that use oxygen for cellular respiration). The citric acid cycle is named after citrate or citric acid, a tricarboxylic acid that is both consumed and regenerated through this pathway. The citric acid cycle was discovered in 1937 by Hans Adolf Krebs while he worked at the University of Sheffield in England (PMID: 16746382). Krebs received the Nobel Prize for his discovery in 1953. Krebs’ extensive work on this pathway is also why the citric acid or TCA cycle is often referred to as the Krebs cycle. Metabolically, the citric acid cycle allows the release of energy (ultimately in the form of ATP) from carbohydrates, fats, and proteins through the oxidation of acetyl-CoA. The citric acid cycle also produces CO2, the precursors for several amino acids (aspartate, asparagine, glutamine, proline) and NADH – all of which are used in other important metabolic pathways, such as amino acid synthesis and oxidative phosphorylation (OxPhos). The net yield of one “turn” of the TCA cycle in terms of energy-containing compounds is one GTP, one FADH2, and three NADH molecules. The NADH molecules are used in oxidative phosphorylation to generate ATP. In eukaryotes, the citric acid cycle occurs in the mitochondrial matrix. In prokaryotes, the citric acid cycle occurs in the cytoplasm. In eukaryotes, the citric acid or TCA cycle has a total of 10 steps that are mediated by 8 different enzymes. Key to the whole cycle is the availability of acetyl-CoA. One of the primary sources of acetyl-CoA is from the breakdown of glucose (and other sugars) by glycolysis. This process generates pyruvate. Pyruvate is decarboxylated by pyruvate dehydrogenase to generate acetyl-CoA. The citric acid cycle begins with acetyl-CoA transferring its two-carbon acetyl group to the four-carbon acceptor compound (oxaloacetate) to form a six-carbon compound (citrate) through the enzyme citrate synthase. The resulting citrate is then converted to cis-aconitate and then isocitrate via the enzyme aconitase. The resulting isocitrate then combines with NAD+ to form oxalosuccinate and NADH, which is then converted into alpha-ketoglutarate (and CO2) through the action of the enzyme known as isocitrate dehydrogenase. The resulting alpha-ketoglutarate combines with NAD+ and CoA-SH to produce succinyl-CoA, NADH, and CO2. This step is mediated by the enzyme alpha-ketoglutarate dehydrogenase. The resulting succinyl-CoA combines with GDP and organic phosphate to produce succinate, CoA-SH, and GTP. This phosphorylation reaction is performed by succinyl-CoA synthase. The resulting succinate then combines with ubiquinone to produce two compounds, fumarate and ubiquinol through the action of the enzyme succinate dehydrogenase. The resulting fumarate is then hydrated by the enzyme known as fumarase to produce malate. The resulting malate is oxidized via NAD+ to produce oxaloacetate and NADH. This oxidation reaction is performed by malate dehydrogenase. The resulting oxaloacetate can then combine with acetyl-CoA and the TCA reaction cycle begins again. Overall, in the citric acid cycle, the starting six-carbon citrate molecule loses two carboxyl groups as CO2, leading to the production of a four-carbon oxaloacetate. The two-carbon acetyl-CoA that is the “fuel” for the TCA cycle can be generated by several metabolic pathways including glucose metabolism, fatty acid oxidation, and the metabolism of amino acids. The overall reaction for the citric acid cycle is as follows: acetyl-CoA + 3 NAD+ + FAD + GDP + P + 2H2O = CoA-SH + 3NADH + FADH2 + 3H+ + GTP + 2CO2. Many molecules in the citric acid cycle serve as key precursors for other molecules needed by cells. The citrate generated via the citric acid cycle can serve as an intermediate for fatty acid synthesis; alpha-ketoglutarate can serve as a precursor for glutamate, proline, and arginine; oxaloacetate can serve as a precursor for aspartate and asparagine; succinyl-CoA can serve as a precursor for porphyrins; and acetyl-CoA can serve as a precursor fatty acids, cholesterol, vitamin D, and various steroid hormones. There are several variations to the citric acid cycle that are known. Interestingly, most of the variation lies with the step involving succinyl-CoA production or conversion. Humans and other animals have two different types of succinyl-CoA synthetases. One produces GTP from GDP, while the other produces ATP from ADP (PMID: 9765291). On the other hand, plants have a succinyl-CoA synthetase that produces ATP (ADP-forming succinyl-CoA synthetase) (Jones RC, Buchanan BB, Gruissem W. (2000). Biochemistry & molecular biology of plants (1st ed.). Rockville, Md: American Society of Plant Physiologists. ISBN 0-943088-39-9.). In certain acetate-producing bacteria, such as Acetobacter aceti, an enzyme known as succinyl-CoA:acetate CoA-transferase performs this conversion (PMID: 18502856) while in Helicobacter pylori succinyl-CoA:acetoacetate CoA-transferase is responsible for this reaction (PMID: 9325289). The citric acid cycle is regulated in a number of ways but the primary mechanism is by product inhibition. For instance, NADH inhibits pyruvate dehydrogenase, isocitrate dehydrogenase, alpha-ketoglutarate dehydrogenase, and citrate synthase. Acetyl-CoA inhibits pyruvate dehydrogenase, while succinyl-CoA inhibits alpha-ketoglutarate dehydrogenase and citrate synthase. Additionally, ATP inhibits citrate synthase and alpha-ketoglutarate dehydrogenase. Calcium is another important regulator of the citric acid cycle. In particular, it activates pyruvate dehydrogenase phosphatase, which then activates pyruvate dehydrogenase. Calcium also activates isocitrate dehydrogenase and alpha-ketoglutarate dehydrogenase (PMID: 171557).
|
Metabolite
Metabolic
|
|
|
SMP0126206 |
Cortisonee acetate Action Action PathwayHomo sapiens
|
Metabolite
Drug Action
|
|
Showing 346061 -
346070 of 364487 pathways