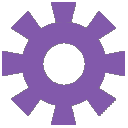
Browsing Pathways
Showing 348291 -
348300 of 605359 pathways
PathBank ID | Pathway Name and Description | Pathway Class | Chemical Compounds | Proteins |
---|---|---|---|---|
SMP0401620![]() |
NAD SalvageEscherichia coli K-12
NAD molecules have a relatively short half-life. NAD can be degraded by enzymes, and the degraded NAD molecule can be recouped by NAD salvage cycles. NAD salvage cycles can be used for recycling degraded NAD products such as nicotinamide and nicotinamide D-ribonucleotide. NAD salvage cycles can also be used for absorption of exogenous NAD+. NAD reacts spontaneously with water resulting in the release of hydrogen ion, AMP and beta-nicotinamide D-ribonucleotide. This enzyme can either interact spontaneously with water resulting in the release of D-ribofuranose 5-phosphate, hydrogen ion and Nacinamide. On the other hand beta-nicotinamide D-ribonucleotide can also react with water through NMN amidohydrolase resulting in ammonium, and Nicotinate beta-D-ribonucleotide. Also it can interact with water spontaneously resulting in the release of phosphate resulting in a Nicotinamide riboside. Niacinamide interacts with water through a nicotinamidase resulting in a release of ammonium and nicotinic acid. Nicotinic acid interacts with water and phosphoribosyl pyrophosphate through an ATP driven nicotinate phosphoribosyltransferase resulting in the release of ADP, pyrophosphate and phosphate and nicotinate beta-D-ribonucleotide. Nicotinamide riboside interacts with an ATP driven NadR DNA-binding transcriptional repressor and NMN adenylyltransferase (Escherichia coli) resulting in a ADP, hydrogen ion and beta-nicotinamide D-ribonucleotide. The latter interacts with ATP and hydrogen ions through NadR DNA-binding transcriptional repressor and NMN adenylyltransferase resulting in pyrophosphate and NAD. Nicotinate beta-D-ribonucleotide is adenylated through the interaction with ATP and a hydrogen ion through a nicotinate-mononucleotide adenylyltransferase resulting in pyrophosphate and Nicotinic acid adenine dinucleotide. Nicotinic acid adenine dinucleotide interacts with L-glutamine and water through an ATP driven NAD synthease, NH3-dependent resulting in AMP, pyrophosphate, hydrogen ion, L-glutamic acid and NAD.
|
Metabolite
Metabolic
|
|
|
SMP0295415 |
Cardiolipin Biosynthesis CL(18:2(9Z,12Z)/18:2(9Z,12Z)/14:0/20:3(11Z,14Z,17Z))Mus musculus
Cardiolipin (CL) is an important component of the inner mitochondrial membrane where it constitutes about 20% of the total lipid composition. It is essential for the optimal function of numerous enzymes that are involved in mitochondrial energy metabolism (Wikipedia). Cardiolipin biosynthesis occurs mainly in the mitochondria, but there also exists an alternative synthesis route for CDP-diacylglycerol that takes place in the endoplasmic reticulum. This second route may supplement this pathway. All membrane-localized enzymes are coloured dark green in the image. First, dihydroxyacetone phosphate (or glycerone phosphate) from glycolysis is used by the cytosolic enzyme glycerol-3-phosphate dehydrogenase [NAD(+)] to synthesize sn-glycerol 3-phosphate. Second, the mitochondrial outer membrane enzyme glycerol-3-phosphate acyltransferase esterifies an acyl-group to the sn-1 position of sn-glycerol 3-phosphate to form 1-acyl-sn-glycerol 3-phosphate (lysophosphatidic acid or LPA). Third, the enzyme 1-acyl-sn-glycerol-3-phosphate acyltransferase converts LPA into phosphatidic acid (PA or 1,2-diacyl-sn-glycerol 3-phosphate) by esterifying an acyl-group to the sn-2 position of the glycerol backbone. PA is then transferred to the inner mitochondrial membrane to continue cardiolipin synthesis. Fourth, magnesium-dependent phosphatidate cytidylyltransferase catalyzes the conversion of PA into CDP-diacylglycerol. Fifth, CDP-diacylglycerol--glycerol-3-phosphate 3-phosphatidyltransferase synthesizes phosphatidylglycerophosphate (PGP). Sixth, phosphatidylglycerophosphatase and protein-tyrosine phosphatase dephosphorylates PGP to form phosphatidylglycerol (PG). Last, cardiolipin synthase catalyzes the synthesis of cardiolipin by transferring a phosphatidyl group from a second CDP-diacylglycerol to PG. It requires a divalent metal cation cofactor.
|
Metabolite
Metabolic
|
|
|
SMP0295403 |
Cardiolipin Biosynthesis CL(18:2(9Z,12Z)/18:2(9Z,12Z)/14:0/14:0)Mus musculus
Cardiolipin (CL) is an important component of the inner mitochondrial membrane where it constitutes about 20% of the total lipid composition. It is essential for the optimal function of numerous enzymes that are involved in mitochondrial energy metabolism (Wikipedia). Cardiolipin biosynthesis occurs mainly in the mitochondria, but there also exists an alternative synthesis route for CDP-diacylglycerol that takes place in the endoplasmic reticulum. This second route may supplement this pathway. All membrane-localized enzymes are coloured dark green in the image. First, dihydroxyacetone phosphate (or glycerone phosphate) from glycolysis is used by the cytosolic enzyme glycerol-3-phosphate dehydrogenase [NAD(+)] to synthesize sn-glycerol 3-phosphate. Second, the mitochondrial outer membrane enzyme glycerol-3-phosphate acyltransferase esterifies an acyl-group to the sn-1 position of sn-glycerol 3-phosphate to form 1-acyl-sn-glycerol 3-phosphate (lysophosphatidic acid or LPA). Third, the enzyme 1-acyl-sn-glycerol-3-phosphate acyltransferase converts LPA into phosphatidic acid (PA or 1,2-diacyl-sn-glycerol 3-phosphate) by esterifying an acyl-group to the sn-2 position of the glycerol backbone. PA is then transferred to the inner mitochondrial membrane to continue cardiolipin synthesis. Fourth, magnesium-dependent phosphatidate cytidylyltransferase catalyzes the conversion of PA into CDP-diacylglycerol. Fifth, CDP-diacylglycerol--glycerol-3-phosphate 3-phosphatidyltransferase synthesizes phosphatidylglycerophosphate (PGP). Sixth, phosphatidylglycerophosphatase and protein-tyrosine phosphatase dephosphorylates PGP to form phosphatidylglycerol (PG). Last, cardiolipin synthase catalyzes the synthesis of cardiolipin by transferring a phosphatidyl group from a second CDP-diacylglycerol to PG. It requires a divalent metal cation cofactor.
|
Metabolite
Metabolic
|
|
|
SMP0295396 |
Cardiolipin Biosynthesis CL(18:2(9Z,12Z)/18:2(9Z,11Z)/20:4(5Z,8Z,11Z,14Z)/16:1(9Z))Mus musculus
Cardiolipin (CL) is an important component of the inner mitochondrial membrane where it constitutes about 20% of the total lipid composition. It is essential for the optimal function of numerous enzymes that are involved in mitochondrial energy metabolism (Wikipedia). Cardiolipin biosynthesis occurs mainly in the mitochondria, but there also exists an alternative synthesis route for CDP-diacylglycerol that takes place in the endoplasmic reticulum. This second route may supplement this pathway. All membrane-localized enzymes are coloured dark green in the image. First, dihydroxyacetone phosphate (or glycerone phosphate) from glycolysis is used by the cytosolic enzyme glycerol-3-phosphate dehydrogenase [NAD(+)] to synthesize sn-glycerol 3-phosphate. Second, the mitochondrial outer membrane enzyme glycerol-3-phosphate acyltransferase esterifies an acyl-group to the sn-1 position of sn-glycerol 3-phosphate to form 1-acyl-sn-glycerol 3-phosphate (lysophosphatidic acid or LPA). Third, the enzyme 1-acyl-sn-glycerol-3-phosphate acyltransferase converts LPA into phosphatidic acid (PA or 1,2-diacyl-sn-glycerol 3-phosphate) by esterifying an acyl-group to the sn-2 position of the glycerol backbone. PA is then transferred to the inner mitochondrial membrane to continue cardiolipin synthesis. Fourth, magnesium-dependent phosphatidate cytidylyltransferase catalyzes the conversion of PA into CDP-diacylglycerol. Fifth, CDP-diacylglycerol--glycerol-3-phosphate 3-phosphatidyltransferase synthesizes phosphatidylglycerophosphate (PGP). Sixth, phosphatidylglycerophosphatase and protein-tyrosine phosphatase dephosphorylates PGP to form phosphatidylglycerol (PG). Last, cardiolipin synthase catalyzes the synthesis of cardiolipin by transferring a phosphatidyl group from a second CDP-diacylglycerol to PG. It requires a divalent metal cation cofactor.
|
Metabolite
Metabolic
|
|
|
SMP0401608![]() |
Mannose MetabolismEscherichia coli K-12
Escherichia coli can utilize D-mannose for its sole carbon and energy source. Alpha-D-mannose is introduced into the cytoplasm through a mannose PTS permease. A phosphotransferase system (PTS) takes up mannose producing D-mannose-6-phosphate which is then converted to D-fructose-6-phosphate via an isomerase. D-fructose-6-phosphate is an intermediate of glycolysis and can enter the pathways of metabolism. The first two enzymes in the pathway catalyze isomerizations that interconvert phosphorylated aldohexoses (β-D-glucose-6-phosphate, D-mannose-6-phosphate) and phosphorylated ketohexoses (D-fructose-6-phosphate). The reaction catalyzed by mannose-6-phosphate isomerase that produces D-mannose-6-phosphate is the first committed step in the biosynthesis of the activated mannose donor GDP-α-D-mannose. D-mannose-6-phosphate is then converted to GDP-D-mannose by the interaction of phosphomannomutase and mannose-1-phosphate guanylyltransferase. GDP-D-mannose produces GDP-L-fucose beginning with the dehydration to GDP-4-dehydro-6-deoxy-D-mannose. GDP-fucose is synthesized by a two step epimerase and reductase of GDP-4-dehydro-6-deoxy-D-mannose. L-fucose then enters the colanic acid building blocks biosynthesis pathway.
|
Metabolite
Metabolic
|
||
SMP0401615![]() |
D-Allulose DegradationEscherichia coli O157:H7
D-allose can be used as source of carbon for E.coli. D-allose is imported into E.coli by D-allose ABC transporter without phosphorylation. Allose-6-phosphate isomerase and allulose-6-phosphate 3-epimerase catalyze the remaining reactions resulting in D-allulose 6 phosphate and Beta-D-fructofuranose 6-phosphate respectively. Once Beta D fructofuranose 6-phosphate is synthesized, it can be used in the glycolysis and pyruvatedehydrogenase pathway.
|
Metabolite
Metabolic
|
||
SMP0391906![]() |
Cysteine BiosynthesisCitrobacter youngae ATCC 29220
The pathway of cysteine biosynthesis is a two-step conversion starting from L-serine and yielding L-cysteine. L-serine biosynthesis is shown for context. L-cysteine can also be synthesized from sulfate derivatives. The process through L-serine involves a serine acetyltransferase that produces a O-acetylserine which reacts together with hydrogen sulfide through a cysteine synthase complex in order to produce L-cysteine and acetic acid. Hydrogen sulfide is produced from a sulfate. Sulfate reacts with sulfate adenylyltransferase to produce adenosine phosphosulfate. This compound in turn is phosphorylated through a adenylyl-sulfate kinase into a phosphoadenosine phosphosulfate which in turn reacts with a phosphoadenosine phosphosulfate reductase to produce a sulfite. The sulfite reacts with a sulfite reductase to produce the hydrogen sulfide. This pathway shows the second step of cysteine biosynthesis (at genetic level). Both cysteine synthase isozymes undergo the positive control by the cysteine-responsive transcription factor CysB. Only cysteine synthase A (CysK) forms a complex with serine acetyltransferase and it is the only cysteine synthase that is required for cell viability with cysteine-free medium. Cysteine synthases may also work as the sulfur scavenging systemfor sulfur starvation by taking sulfur off of L-cysteine.
|
Metabolite
Metabolic
|
|
|
SMP0400689![]() |
D-Glucarate and D-Galactarate DegradationCitrobacter youngae ATCC 29220
Galactarate is a naturally occurring dicarboxylic acid analog of D-galactose. E. coli can use both diacid sugars galactarate and D-glucarate as the sole source of carbon for growth. The initial step in the degradation of galactarate is its dehydration to 5-dehydro-4-deoxy-D-glucarate(2--) by galactarate dehydratase. Glucaric acid can also be dehydrated by a glucarate dehydratase resulting in water and 5-dehydro-4-deoxy-D-glucarate(2--). The 5-dehydro-4-deoxy-D-glucarate(2--) is then metabolized by a alpha-dehydro-beta-deoxy-D-glucarate aldolase resulting in pyruvic acid and a tartonate semialdehyde. Pyruvic acid interacts with coenzyme A through a NAD driven Pyruvate dehydrogenase complex resulting in a carbon dioxide, an NADH and an acetyl-CoA. The tartronate semialdehyde interacts with a hydrogen ion through a NADPH driven tartronate semialdehyde reductase resulting in a NADP and a glyceric acid. The glyceric acid is phosphorylated by an ATP-driven glycerate kinase 2 resulting in an ADP, a hydrogen ion and a 2-phosphoglyceric acid. The latter compound is dehydrated by an enolase resulting in the release of water and a phosphoenolpyruvic acid. The phosphoenolpyruvic acid interacts with a hydrogen ion through an ADP driven pyruvate kinase resulting in an ATP and a pyruvic acid. The pyruvic acid then interacts with water and an ATP through a phosphoenolpyruvate synthetase resulting in the release of a hydrogen ion, a phosphate, an AMP and a Phosphoenolpyruvic acid.
|
Metabolite
Metabolic
|
|
|
SMP0294133 |
Cardiolipin Biosynthesis CL(18:2(9Z,11Z)/18:2(9Z,11Z)/20:4(5Z,8Z,11Z,14Z)/18:2(9Z,12Z))Mus musculus
Cardiolipin (CL) is an important component of the inner mitochondrial membrane where it constitutes about 20% of the total lipid composition. It is essential for the optimal function of numerous enzymes that are involved in mitochondrial energy metabolism (Wikipedia). Cardiolipin biosynthesis occurs mainly in the mitochondria, but there also exists an alternative synthesis route for CDP-diacylglycerol that takes place in the endoplasmic reticulum. This second route may supplement this pathway. All membrane-localized enzymes are coloured dark green in the image. First, dihydroxyacetone phosphate (or glycerone phosphate) from glycolysis is used by the cytosolic enzyme glycerol-3-phosphate dehydrogenase [NAD(+)] to synthesize sn-glycerol 3-phosphate. Second, the mitochondrial outer membrane enzyme glycerol-3-phosphate acyltransferase esterifies an acyl-group to the sn-1 position of sn-glycerol 3-phosphate to form 1-acyl-sn-glycerol 3-phosphate (lysophosphatidic acid or LPA). Third, the enzyme 1-acyl-sn-glycerol-3-phosphate acyltransferase converts LPA into phosphatidic acid (PA or 1,2-diacyl-sn-glycerol 3-phosphate) by esterifying an acyl-group to the sn-2 position of the glycerol backbone. PA is then transferred to the inner mitochondrial membrane to continue cardiolipin synthesis. Fourth, magnesium-dependent phosphatidate cytidylyltransferase catalyzes the conversion of PA into CDP-diacylglycerol. Fifth, CDP-diacylglycerol--glycerol-3-phosphate 3-phosphatidyltransferase synthesizes phosphatidylglycerophosphate (PGP). Sixth, phosphatidylglycerophosphatase and protein-tyrosine phosphatase dephosphorylates PGP to form phosphatidylglycerol (PG). Last, cardiolipin synthase catalyzes the synthesis of cardiolipin by transferring a phosphatidyl group from a second CDP-diacylglycerol to PG. It requires a divalent metal cation cofactor.
|
Metabolite
Metabolic
|
|
|
SMP0294131 |
Cardiolipin Biosynthesis CL(18:2(9Z,11Z)/18:2(9Z,11Z)/20:4(5Z,8Z,11Z,14Z)/18:1(9Z))Mus musculus
Cardiolipin (CL) is an important component of the inner mitochondrial membrane where it constitutes about 20% of the total lipid composition. It is essential for the optimal function of numerous enzymes that are involved in mitochondrial energy metabolism (Wikipedia). Cardiolipin biosynthesis occurs mainly in the mitochondria, but there also exists an alternative synthesis route for CDP-diacylglycerol that takes place in the endoplasmic reticulum. This second route may supplement this pathway. All membrane-localized enzymes are coloured dark green in the image. First, dihydroxyacetone phosphate (or glycerone phosphate) from glycolysis is used by the cytosolic enzyme glycerol-3-phosphate dehydrogenase [NAD(+)] to synthesize sn-glycerol 3-phosphate. Second, the mitochondrial outer membrane enzyme glycerol-3-phosphate acyltransferase esterifies an acyl-group to the sn-1 position of sn-glycerol 3-phosphate to form 1-acyl-sn-glycerol 3-phosphate (lysophosphatidic acid or LPA). Third, the enzyme 1-acyl-sn-glycerol-3-phosphate acyltransferase converts LPA into phosphatidic acid (PA or 1,2-diacyl-sn-glycerol 3-phosphate) by esterifying an acyl-group to the sn-2 position of the glycerol backbone. PA is then transferred to the inner mitochondrial membrane to continue cardiolipin synthesis. Fourth, magnesium-dependent phosphatidate cytidylyltransferase catalyzes the conversion of PA into CDP-diacylglycerol. Fifth, CDP-diacylglycerol--glycerol-3-phosphate 3-phosphatidyltransferase synthesizes phosphatidylglycerophosphate (PGP). Sixth, phosphatidylglycerophosphatase and protein-tyrosine phosphatase dephosphorylates PGP to form phosphatidylglycerol (PG). Last, cardiolipin synthase catalyzes the synthesis of cardiolipin by transferring a phosphatidyl group from a second CDP-diacylglycerol to PG. It requires a divalent metal cation cofactor.
|
Metabolite
Metabolic
|
|
Showing 348291 -
348300 of 348699 pathways