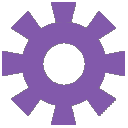
Browsing Pathways
Showing 348341 -
348350 of 605359 pathways
PathBank ID | Pathway Name and Description | Pathway Class | Chemical Compounds | Proteins |
---|---|---|---|---|
SMP0399949![]() |
tRNA ChargingRudanella lutea DSM 19387
This pathway is a compilation of Escherichia coli tRNA charging reactions involving amino acids transported into the cell. The aminoacyl-tRNA synthetase is an enzyme that attaches the appropriate amino acid onto its tRNA by catalyzing the esterification of a specific cognate amino acid or its precursor to one of all its compatible cognate tRNAs to form an aminoacyl-tRNA, which plays an important role in RNA translation. 20 different Aminoacyl-tRNA synthetases can make 20 different types of aa-tRNA for each amino acid according to the genetic code. This process is called "charging" or "loading" the tRNA with amino acid. Ribosome can transfer the amino acid from tRNA to a growing peptide after the tRNA is charged.
|
Metabolite
Metabolic
|
|
|
SMP0293538 |
Cardiolipin Biosynthesis CL(18:1(9Z)/22:5(7Z,10Z,13Z,16Z,19Z)/14:0/20:5(5Z,8Z,11Z,14Z,17Z))Mus musculus
Cardiolipin (CL) is an important component of the inner mitochondrial membrane where it constitutes about 20% of the total lipid composition. It is essential for the optimal function of numerous enzymes that are involved in mitochondrial energy metabolism (Wikipedia). Cardiolipin biosynthesis occurs mainly in the mitochondria, but there also exists an alternative synthesis route for CDP-diacylglycerol that takes place in the endoplasmic reticulum. This second route may supplement this pathway. All membrane-localized enzymes are coloured dark green in the image. First, dihydroxyacetone phosphate (or glycerone phosphate) from glycolysis is used by the cytosolic enzyme glycerol-3-phosphate dehydrogenase [NAD(+)] to synthesize sn-glycerol 3-phosphate. Second, the mitochondrial outer membrane enzyme glycerol-3-phosphate acyltransferase esterifies an acyl-group to the sn-1 position of sn-glycerol 3-phosphate to form 1-acyl-sn-glycerol 3-phosphate (lysophosphatidic acid or LPA). Third, the enzyme 1-acyl-sn-glycerol-3-phosphate acyltransferase converts LPA into phosphatidic acid (PA or 1,2-diacyl-sn-glycerol 3-phosphate) by esterifying an acyl-group to the sn-2 position of the glycerol backbone. PA is then transferred to the inner mitochondrial membrane to continue cardiolipin synthesis. Fourth, magnesium-dependent phosphatidate cytidylyltransferase catalyzes the conversion of PA into CDP-diacylglycerol. Fifth, CDP-diacylglycerol--glycerol-3-phosphate 3-phosphatidyltransferase synthesizes phosphatidylglycerophosphate (PGP). Sixth, phosphatidylglycerophosphatase and protein-tyrosine phosphatase dephosphorylates PGP to form phosphatidylglycerol (PG). Last, cardiolipin synthase catalyzes the synthesis of cardiolipin by transferring a phosphatidyl group from a second CDP-diacylglycerol to PG. It requires a divalent metal cation cofactor.
|
Metabolite
Metabolic
|
|
|
SMP0293531 |
Cardiolipin Biosynthesis CL(18:1(9Z)/22:5(7Z,10Z,13Z,16Z,19Z)/14:0/18:2(9Z,12Z))Mus musculus
Cardiolipin (CL) is an important component of the inner mitochondrial membrane where it constitutes about 20% of the total lipid composition. It is essential for the optimal function of numerous enzymes that are involved in mitochondrial energy metabolism (Wikipedia). Cardiolipin biosynthesis occurs mainly in the mitochondria, but there also exists an alternative synthesis route for CDP-diacylglycerol that takes place in the endoplasmic reticulum. This second route may supplement this pathway. All membrane-localized enzymes are coloured dark green in the image. First, dihydroxyacetone phosphate (or glycerone phosphate) from glycolysis is used by the cytosolic enzyme glycerol-3-phosphate dehydrogenase [NAD(+)] to synthesize sn-glycerol 3-phosphate. Second, the mitochondrial outer membrane enzyme glycerol-3-phosphate acyltransferase esterifies an acyl-group to the sn-1 position of sn-glycerol 3-phosphate to form 1-acyl-sn-glycerol 3-phosphate (lysophosphatidic acid or LPA). Third, the enzyme 1-acyl-sn-glycerol-3-phosphate acyltransferase converts LPA into phosphatidic acid (PA or 1,2-diacyl-sn-glycerol 3-phosphate) by esterifying an acyl-group to the sn-2 position of the glycerol backbone. PA is then transferred to the inner mitochondrial membrane to continue cardiolipin synthesis. Fourth, magnesium-dependent phosphatidate cytidylyltransferase catalyzes the conversion of PA into CDP-diacylglycerol. Fifth, CDP-diacylglycerol--glycerol-3-phosphate 3-phosphatidyltransferase synthesizes phosphatidylglycerophosphate (PGP). Sixth, phosphatidylglycerophosphatase and protein-tyrosine phosphatase dephosphorylates PGP to form phosphatidylglycerol (PG). Last, cardiolipin synthase catalyzes the synthesis of cardiolipin by transferring a phosphatidyl group from a second CDP-diacylglycerol to PG. It requires a divalent metal cation cofactor.
|
Metabolite
Metabolic
|
|
|
SMP0399954![]() |
Chorismate Biosynthesis[Clostridium] citroniae WAL-17108
Chorismate is an intermediate in tyrosine, phenylalanine and tryptophan synthesis and a precursor for folic acid, ubiquinone, enterochelin and menaquinone. Three enzymes catalyze the first step in chorismate biosynthesis. Synthesis may be reduced by feedback inhibition of tyrosine, phenylalanine and tryptophan to the enzymes. The biosynthesis of chorismate starts with D-Erythrose-4-phosphate getting transformed into 3-deoxy-D-arabino-heptulosonate-7-phosphate through a phospho-2-dehydro-3-deoxyheptonate aldolase. This is followed by a 3-dehydroquinate synthase converting the 3-deoxy-D-arabino-heptulosonate-7-phosphate into a 3-dehydroquinate which in turn is conveted to 3-dehydroshikimate through a 3-dehydroquinate dehydratase. At this point 3-dehydroshikimate can be turned into Shikimic acid through 2 different reactions involving Quinate/shikimate dehydrogenase and shikimate dehydrogenase 2. Shikimic acid is phosphorylated by Shikimate kinase 2 into shikimate 3-phosphate. Shikimate 3- phophate and a phosphoenolpyruvic acid are then joined through a 3-phosphoshikimate 1-carboxyvinyltransferase to produce a 5-enoylpyruvyl-shikimate 3-phosphate while releasing a phosphate. This in turn produces our final product Chorismate through a chorismate synthase.
|
Metabolite
Metabolic
|
|
|
SMP0399951![]() |
Chorismate Biosynthesis[Clostridium] symbiosum ATCC 14940
Chorismate is an intermediate in tyrosine, phenylalanine and tryptophan synthesis and a precursor for folic acid, ubiquinone, enterochelin and menaquinone. Three enzymes catalyze the first step in chorismate biosynthesis. Synthesis may be reduced by feedback inhibition of tyrosine, phenylalanine and tryptophan to the enzymes. The biosynthesis of chorismate starts with D-Erythrose-4-phosphate getting transformed into 3-deoxy-D-arabino-heptulosonate-7-phosphate through a phospho-2-dehydro-3-deoxyheptonate aldolase. This is followed by a 3-dehydroquinate synthase converting the 3-deoxy-D-arabino-heptulosonate-7-phosphate into a 3-dehydroquinate which in turn is conveted to 3-dehydroshikimate through a 3-dehydroquinate dehydratase. At this point 3-dehydroshikimate can be turned into Shikimic acid through 2 different reactions involving Quinate/shikimate dehydrogenase and shikimate dehydrogenase 2. Shikimic acid is phosphorylated by Shikimate kinase 2 into shikimate 3-phosphate. Shikimate 3- phophate and a phosphoenolpyruvic acid are then joined through a 3-phosphoshikimate 1-carboxyvinyltransferase to produce a 5-enoylpyruvyl-shikimate 3-phosphate while releasing a phosphate. This in turn produces our final product Chorismate through a chorismate synthase.
|
Metabolite
Metabolic
|
|
|
SMP0399959![]() |
Phenylalanine BiosynthesisRudanella lutea DSM 19387
The phenylalaline biosynthesis pathways is connected with the chorismate biosynthesis pathway. Chorismate biosynthesis produce the chorismate, which further be converted to prephenate by P-protein. Combined with cofactor, H+, prephenate has been further converted to phenylpyruvic acid by P-protein with generated water and carbon dioxide. Phenylalanine transaminase catalyzes phenylpyruvic acid to phenylalaline, and also convert glutamic acid to oxoglutaric acid. Phenylalaline will be further used in phenylalaline metabolism.
|
Metabolite
Metabolic
|
||
SMP0399956![]() |
Cardiolipin Biosynthesis CL(a-15:0/i-15:0/16:0/17:0)Homo sapiens
Cardiolipin (CL) is an important component of the inner mitochondrial membrane where it constitutes about 20% of the total lipid composition. It is essential for the optimal function of numerous enzymes that are involved in mitochondrial energy metabolism (Wikipedia). Cardiolipin biosynthesis occurs mainly in the mitochondria, but there also exists an alternative synthesis route for CDP-diacylglycerol that takes place in the endoplasmic reticulum. This second route may supplement this pathway. All membrane-localized enzymes are coloured dark green in the image. First, dihydroxyacetone phosphate (or glycerone phosphate) from glycolysis is used by the cytosolic enzyme glycerol-3-phosphate dehydrogenase [NAD(+)] to synthesize sn-glycerol 3-phosphate. Second, the mitochondrial outer membrane enzyme glycerol-3-phosphate acyltransferase esterifies an acyl-group to the sn-1 position of sn-glycerol 3-phosphate to form 1-acyl-sn-glycerol 3-phosphate (lysophosphatidic acid or LPA). Third, the enzyme 1-acyl-sn-glycerol-3-phosphate acyltransferase converts LPA into phosphatidic acid (PA or 1,2-diacyl-sn-glycerol 3-phosphate) by esterifying an acyl-group to the sn-2 position of the glycerol backbone. PA is then transferred to the inner mitochondrial membrane to continue cardiolipin synthesis. Fourth, magnesium-dependent phosphatidate cytidylyltransferase catalyzes the conversion of PA into CDP-diacylglycerol. Fifth, CDP-diacylglycerol--glycerol-3-phosphate 3-phosphatidyltransferase synthesizes phosphatidylglycerophosphate (PGP). Sixth, phosphatidylglycerophosphatase and protein-tyrosine phosphatase dephosphorylates PGP to form phosphatidylglycerol (PG). Last, cardiolipin synthase catalyzes the synthesis of cardiolipin by transferring a phosphatidyl group from a second CDP-diacylglycerol to PG. It requires a divalent metal cation cofactor.
|
Metabolite
Metabolic
|
|
|
SMP0399964![]() |
Cardiolipin Biosynthesis CL(a-15:0/i-15:0/16:0/18:0)Homo sapiens
Cardiolipin (CL) is an important component of the inner mitochondrial membrane where it constitutes about 20% of the total lipid composition. It is essential for the optimal function of numerous enzymes that are involved in mitochondrial energy metabolism (Wikipedia). Cardiolipin biosynthesis occurs mainly in the mitochondria, but there also exists an alternative synthesis route for CDP-diacylglycerol that takes place in the endoplasmic reticulum. This second route may supplement this pathway. All membrane-localized enzymes are coloured dark green in the image. First, dihydroxyacetone phosphate (or glycerone phosphate) from glycolysis is used by the cytosolic enzyme glycerol-3-phosphate dehydrogenase [NAD(+)] to synthesize sn-glycerol 3-phosphate. Second, the mitochondrial outer membrane enzyme glycerol-3-phosphate acyltransferase esterifies an acyl-group to the sn-1 position of sn-glycerol 3-phosphate to form 1-acyl-sn-glycerol 3-phosphate (lysophosphatidic acid or LPA). Third, the enzyme 1-acyl-sn-glycerol-3-phosphate acyltransferase converts LPA into phosphatidic acid (PA or 1,2-diacyl-sn-glycerol 3-phosphate) by esterifying an acyl-group to the sn-2 position of the glycerol backbone. PA is then transferred to the inner mitochondrial membrane to continue cardiolipin synthesis. Fourth, magnesium-dependent phosphatidate cytidylyltransferase catalyzes the conversion of PA into CDP-diacylglycerol. Fifth, CDP-diacylglycerol--glycerol-3-phosphate 3-phosphatidyltransferase synthesizes phosphatidylglycerophosphate (PGP). Sixth, phosphatidylglycerophosphatase and protein-tyrosine phosphatase dephosphorylates PGP to form phosphatidylglycerol (PG). Last, cardiolipin synthase catalyzes the synthesis of cardiolipin by transferring a phosphatidyl group from a second CDP-diacylglycerol to PG. It requires a divalent metal cation cofactor.
|
Metabolite
Metabolic
|
|
|
SMP0400117![]() |
Cysteine BiosynthesisFusobacterium nucleatum subsp. animalis 7_1
The pathway of cysteine biosynthesis is a two-step conversion starting from L-serine and yielding L-cysteine. L-serine biosynthesis is shown for context. L-cysteine can also be synthesized from sulfate derivatives. The process through L-serine involves a serine acetyltransferase that produces a O-acetylserine which reacts together with hydrogen sulfide through a cysteine synthase complex in order to produce L-cysteine and acetic acid. Hydrogen sulfide is produced from a sulfate. Sulfate reacts with sulfate adenylyltransferase to produce adenosine phosphosulfate. This compound in turn is phosphorylated through a adenylyl-sulfate kinase into a phosphoadenosine phosphosulfate which in turn reacts with a phosphoadenosine phosphosulfate reductase to produce a sulfite. The sulfite reacts with a sulfite reductase to produce the hydrogen sulfide. This pathway shows the second step of cysteine biosynthesis (at genetic level). Both cysteine synthase isozymes undergo the positive control by the cysteine-responsive transcription factor CysB. Only cysteine synthase A (CysK) forms a complex with serine acetyltransferase and it is the only cysteine synthase that is required for cell viability with cysteine-free medium. Cysteine synthases may also work as the sulfur scavenging systemfor sulfur starvation by taking sulfur off of L-cysteine.
|
Metabolite
Metabolic
|
|
|
SMP0400119![]() |
Tryptophan MetabolismVeillonella atypica ACS-049-V-Sch6
The biosynthesis of L-tryptophan begins with L-glutamine interacting with a chorismate through a anthranilate synthase which results in a L-glutamic acid, a pyruvic acid, a hydrogen ion and a 2-aminobenzoic acid. The aminobenzoic acid interacts with a phosphoribosyl pyrophosphate through an anthranilate synthase component II resulting in a pyrophosphate and a N-(5-phosphoribosyl)-anthranilate. The latter compound is then metabolized by an indole-3-glycerol phosphate synthase / phosphoribosylanthranilate isomerase resulting in a 1-(o-carboxyphenylamino)-1-deoxyribulose 5'-phosphate. This compound then interacts with a hydrogen ion through a indole-3-glycerol phosphate synthase / phosphoribosylanthranilate isomerase resulting in the release of carbon dioxide, a water molecule and a (1S,2R)-1-C-(indol-3-yl)glycerol 3-phosphate. The latter compound then interacts with a D-glyceraldehyde 3-phosphate and an Indole. The indole interacts with an L-serine through a tryptophan synthase, β subunit dimer resulting in a water molecule and an L-tryptophan.
The metabolism of L-tryptophan starts with L-tryptophan being dehydrogenated by a tryptophanase / L-cysteine desulfhydrase resulting in the release of a hydrogen ion, an Indole and a 2-aminoacrylic acid. The latter compound is isomerized into a 2-iminopropanoate. This compound then interacts with a water molecule and a hydrogen ion spontaneously resulting in the release of an Ammonium and a pyruvic acid. The pyruvic acid then interacts with a coenzyme A through a NAD driven pyruvate dehydrogenase complex resulting in the release of a NADH, a carbon dioxide and an Acetyl-CoA
|
Metabolite
Metabolic
|
|
Showing 348341 -
348350 of 348877 pathways