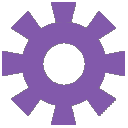
Browsing Pathways
Showing 348431 -
348440 of 605359 pathways
PathBank ID | Pathway Name and Description | Pathway Class | Chemical Compounds | Proteins |
---|---|---|---|---|
SMP0398987![]() |
Gluconeogenesis from L-Malic AcidMethylobacterium mesophilicum SR1.6/6
Gluconeogenesis from L-malic acid starts from the introduction of L-malic acid into cytoplasm either through a C4 dicarboxylate / orotate:H+ symporter or a dicarboxylate transporter (succinic acid antiporter). L-malic acid is then metabolized through 3 possible ways: NAD driven malate dehydrogenase resulting in oxalacetic acid, NADP driven malate dehydrogenase B resulting pyruvic acid or malate dehydrogenase, NAD-requiring resulting in pyruvic acid.
Oxalacetic acid is processed by phosphoenolpyruvate carboxykinase (ATP driven) while pyruvic acid is processed by phosphoenolpyruvate synthetase resulting in phosphoenolpyruvic acid. This compound is dehydrated by enolase resulting in an 2-phosphoglyceric acid which is then isomerized by 2,3-bisphosphoglycerate-independent phosphoglycerate mutase resulting in a 3-phosphoglyceric acid which is phosphorylated by an ATP driven phosphoglycerate kinase resulting in a glyceric acid 1,3-biphosphate. This compound undergoes an NADH driven glyceraldehyde 3-phosphate dehydrogenase reaction resulting in a D-Glyceraldehyde 3-phosphate which is first isomerized into dihydroxyacetone phosphate through an triosephosphate isomerase. D-glyceraldehyde 3-phosphate and Dihydroxyacetone phosphate react through a fructose biphosphate aldolase protein complex resulting in a fructose 1,6-biphosphate. Fructose 1,6-biphosphateis is metabolized by a fructose-1,6-bisphosphatase resulting in a Beta-D-fructofuranose 6-phosphate which is then isomerized into a Beta-D-glucose 6-phosphate through a glucose-6-phosphate isomerase.
|
Metabolite
Metabolic
|
|
|
SMP0292952 |
Cardiolipin Biosynthesis CL(18:1(9Z)/20:3(11Z,14Z,17Z)/22:5(7Z,10Z,13Z,16Z,19Z)/18:4(6Z,9Z,12Z,15Z))Mus musculus
Cardiolipin (CL) is an important component of the inner mitochondrial membrane where it constitutes about 20% of the total lipid composition. It is essential for the optimal function of numerous enzymes that are involved in mitochondrial energy metabolism (Wikipedia). Cardiolipin biosynthesis occurs mainly in the mitochondria, but there also exists an alternative synthesis route for CDP-diacylglycerol that takes place in the endoplasmic reticulum. This second route may supplement this pathway. All membrane-localized enzymes are coloured dark green in the image. First, dihydroxyacetone phosphate (or glycerone phosphate) from glycolysis is used by the cytosolic enzyme glycerol-3-phosphate dehydrogenase [NAD(+)] to synthesize sn-glycerol 3-phosphate. Second, the mitochondrial outer membrane enzyme glycerol-3-phosphate acyltransferase esterifies an acyl-group to the sn-1 position of sn-glycerol 3-phosphate to form 1-acyl-sn-glycerol 3-phosphate (lysophosphatidic acid or LPA). Third, the enzyme 1-acyl-sn-glycerol-3-phosphate acyltransferase converts LPA into phosphatidic acid (PA or 1,2-diacyl-sn-glycerol 3-phosphate) by esterifying an acyl-group to the sn-2 position of the glycerol backbone. PA is then transferred to the inner mitochondrial membrane to continue cardiolipin synthesis. Fourth, magnesium-dependent phosphatidate cytidylyltransferase catalyzes the conversion of PA into CDP-diacylglycerol. Fifth, CDP-diacylglycerol--glycerol-3-phosphate 3-phosphatidyltransferase synthesizes phosphatidylglycerophosphate (PGP). Sixth, phosphatidylglycerophosphatase and protein-tyrosine phosphatase dephosphorylates PGP to form phosphatidylglycerol (PG). Last, cardiolipin synthase catalyzes the synthesis of cardiolipin by transferring a phosphatidyl group from a second CDP-diacylglycerol to PG. It requires a divalent metal cation cofactor.
|
Metabolite
Metabolic
|
|
|
SMP0293034 |
Cardiolipin Biosynthesis CL(18:1(9Z)/20:4(5Z,8Z,11Z,14Z)/18:1(9Z)/16:1(9Z))Mus musculus
Cardiolipin (CL) is an important component of the inner mitochondrial membrane where it constitutes about 20% of the total lipid composition. It is essential for the optimal function of numerous enzymes that are involved in mitochondrial energy metabolism (Wikipedia). Cardiolipin biosynthesis occurs mainly in the mitochondria, but there also exists an alternative synthesis route for CDP-diacylglycerol that takes place in the endoplasmic reticulum. This second route may supplement this pathway. All membrane-localized enzymes are coloured dark green in the image. First, dihydroxyacetone phosphate (or glycerone phosphate) from glycolysis is used by the cytosolic enzyme glycerol-3-phosphate dehydrogenase [NAD(+)] to synthesize sn-glycerol 3-phosphate. Second, the mitochondrial outer membrane enzyme glycerol-3-phosphate acyltransferase esterifies an acyl-group to the sn-1 position of sn-glycerol 3-phosphate to form 1-acyl-sn-glycerol 3-phosphate (lysophosphatidic acid or LPA). Third, the enzyme 1-acyl-sn-glycerol-3-phosphate acyltransferase converts LPA into phosphatidic acid (PA or 1,2-diacyl-sn-glycerol 3-phosphate) by esterifying an acyl-group to the sn-2 position of the glycerol backbone. PA is then transferred to the inner mitochondrial membrane to continue cardiolipin synthesis. Fourth, magnesium-dependent phosphatidate cytidylyltransferase catalyzes the conversion of PA into CDP-diacylglycerol. Fifth, CDP-diacylglycerol--glycerol-3-phosphate 3-phosphatidyltransferase synthesizes phosphatidylglycerophosphate (PGP). Sixth, phosphatidylglycerophosphatase and protein-tyrosine phosphatase dephosphorylates PGP to form phosphatidylglycerol (PG). Last, cardiolipin synthase catalyzes the synthesis of cardiolipin by transferring a phosphatidyl group from a second CDP-diacylglycerol to PG. It requires a divalent metal cation cofactor.
|
Metabolite
Metabolic
|
|
|
SMP0399115![]() |
Threonine BiosynthesisNeisseria flavescens SK114
The biosynthesis of threonine starts with oxalacetic acid interacting with an L-glutamic acid through an aspartate aminotransferase resulting in a oxoglutaric acid and an L-aspartic acid. The latter compound is then phosphorylated by an ATP driven Aspartate kinase resulting in an a release of an ADP and an L-aspartyl-4-phosphate. L-aspartyl-4-phosphate then interacts with a hydrogen ion through an NADPH driven aspartate semialdehyde dehydrogenase resulting in the release of a phosphate, an NADP and a L-aspartate-semialdehyde. The latter compound interacts with a hydrogen ion through a NADPH driven aspartate kinase / homoserine dehydrogenase resulting in the release of an NADP and a L-homoserine. L-homoserine is phosphorylated through an ATP driven homoserine kinase resulting in the release of an ADP, a hydrogen ion and a O-phosphohomoserine. O-phosphohomoserine then interacts with a water molecule and threonine synthase resulting in the release of a phosphate and an L-threonine.
|
Metabolite
Metabolic
|
|
|
SMP0399133![]() |
Isoleucine BiosynthesisNeisseria macacae ATCC 33926
Isoleucine biosynthesis begins with L-threonine from the threonine biosynthesis pathway. L-threonine interacts with threonine dehydratase biosynthetic releasing water, a hydrogen ion and (2Z)-2-aminobut-2-enoate. The latter is isomerized into a 2-iminobutanoate which interacts with water and a hydrogen ion spontaneously, resulting in the release of ammonium and 2-ketobutyric acid. 2-ketobutyric acid reacts with pyruvic acid and hydrogen ions through an acetohydroxybutanoate synthase / acetolactate synthase 2 resulting in carbon dioxide and (S)-2-Aceto-2-hydroxybutanoic acid. (S)-2-Aceto-2-hydroxybutanoic acid is reduced by an NADPH driven acetohydroxy acid isomeroreductase releasing NADP and acetohydroxy acid isomeroreductase. The latter compound is dehydrated by a dihydroxy acid dehydratase resulting in 3-methyl-2-oxovaleric acid. This compound reacts in a reversible reaction with L-glutamic acid through a Branched-chain-amino-acid aminotransferase resulting in oxoglutaric acid and L-isoleucine.
L-isoleucine can also be transported into the cytoplasm through two different methods: a branched chain amino acid ABC transporter or a branched chain amino acid transporter BrnQy.
|
Metabolite
Metabolic
|
|
|
SMP0293041 |
Cardiolipin Biosynthesis CL(18:1(9Z)/20:4(5Z,8Z,11Z,14Z)/18:1(9Z)/20:3(11Z,14Z,17Z))Mus musculus
Cardiolipin (CL) is an important component of the inner mitochondrial membrane where it constitutes about 20% of the total lipid composition. It is essential for the optimal function of numerous enzymes that are involved in mitochondrial energy metabolism (Wikipedia). Cardiolipin biosynthesis occurs mainly in the mitochondria, but there also exists an alternative synthesis route for CDP-diacylglycerol that takes place in the endoplasmic reticulum. This second route may supplement this pathway. All membrane-localized enzymes are coloured dark green in the image. First, dihydroxyacetone phosphate (or glycerone phosphate) from glycolysis is used by the cytosolic enzyme glycerol-3-phosphate dehydrogenase [NAD(+)] to synthesize sn-glycerol 3-phosphate. Second, the mitochondrial outer membrane enzyme glycerol-3-phosphate acyltransferase esterifies an acyl-group to the sn-1 position of sn-glycerol 3-phosphate to form 1-acyl-sn-glycerol 3-phosphate (lysophosphatidic acid or LPA). Third, the enzyme 1-acyl-sn-glycerol-3-phosphate acyltransferase converts LPA into phosphatidic acid (PA or 1,2-diacyl-sn-glycerol 3-phosphate) by esterifying an acyl-group to the sn-2 position of the glycerol backbone. PA is then transferred to the inner mitochondrial membrane to continue cardiolipin synthesis. Fourth, magnesium-dependent phosphatidate cytidylyltransferase catalyzes the conversion of PA into CDP-diacylglycerol. Fifth, CDP-diacylglycerol--glycerol-3-phosphate 3-phosphatidyltransferase synthesizes phosphatidylglycerophosphate (PGP). Sixth, phosphatidylglycerophosphatase and protein-tyrosine phosphatase dephosphorylates PGP to form phosphatidylglycerol (PG). Last, cardiolipin synthase catalyzes the synthesis of cardiolipin by transferring a phosphatidyl group from a second CDP-diacylglycerol to PG. It requires a divalent metal cation cofactor.
|
Metabolite
Metabolic
|
|
|
SMP0293029 |
Cardiolipin Biosynthesis CL(18:1(9Z)/20:4(5Z,8Z,11Z,14Z)/18:0/22:5(4Z,7Z,10Z,13Z,16Z))Mus musculus
Cardiolipin (CL) is an important component of the inner mitochondrial membrane where it constitutes about 20% of the total lipid composition. It is essential for the optimal function of numerous enzymes that are involved in mitochondrial energy metabolism (Wikipedia). Cardiolipin biosynthesis occurs mainly in the mitochondria, but there also exists an alternative synthesis route for CDP-diacylglycerol that takes place in the endoplasmic reticulum. This second route may supplement this pathway. All membrane-localized enzymes are coloured dark green in the image. First, dihydroxyacetone phosphate (or glycerone phosphate) from glycolysis is used by the cytosolic enzyme glycerol-3-phosphate dehydrogenase [NAD(+)] to synthesize sn-glycerol 3-phosphate. Second, the mitochondrial outer membrane enzyme glycerol-3-phosphate acyltransferase esterifies an acyl-group to the sn-1 position of sn-glycerol 3-phosphate to form 1-acyl-sn-glycerol 3-phosphate (lysophosphatidic acid or LPA). Third, the enzyme 1-acyl-sn-glycerol-3-phosphate acyltransferase converts LPA into phosphatidic acid (PA or 1,2-diacyl-sn-glycerol 3-phosphate) by esterifying an acyl-group to the sn-2 position of the glycerol backbone. PA is then transferred to the inner mitochondrial membrane to continue cardiolipin synthesis. Fourth, magnesium-dependent phosphatidate cytidylyltransferase catalyzes the conversion of PA into CDP-diacylglycerol. Fifth, CDP-diacylglycerol--glycerol-3-phosphate 3-phosphatidyltransferase synthesizes phosphatidylglycerophosphate (PGP). Sixth, phosphatidylglycerophosphatase and protein-tyrosine phosphatase dephosphorylates PGP to form phosphatidylglycerol (PG). Last, cardiolipin synthase catalyzes the synthesis of cardiolipin by transferring a phosphatidyl group from a second CDP-diacylglycerol to PG. It requires a divalent metal cation cofactor.
|
Metabolite
Metabolic
|
|
|
SMP0293036 |
Cardiolipin Biosynthesis CL(18:1(9Z)/20:4(5Z,8Z,11Z,14Z)/18:1(9Z)/18:1(9Z))Mus musculus
Cardiolipin (CL) is an important component of the inner mitochondrial membrane where it constitutes about 20% of the total lipid composition. It is essential for the optimal function of numerous enzymes that are involved in mitochondrial energy metabolism (Wikipedia). Cardiolipin biosynthesis occurs mainly in the mitochondria, but there also exists an alternative synthesis route for CDP-diacylglycerol that takes place in the endoplasmic reticulum. This second route may supplement this pathway. All membrane-localized enzymes are coloured dark green in the image. First, dihydroxyacetone phosphate (or glycerone phosphate) from glycolysis is used by the cytosolic enzyme glycerol-3-phosphate dehydrogenase [NAD(+)] to synthesize sn-glycerol 3-phosphate. Second, the mitochondrial outer membrane enzyme glycerol-3-phosphate acyltransferase esterifies an acyl-group to the sn-1 position of sn-glycerol 3-phosphate to form 1-acyl-sn-glycerol 3-phosphate (lysophosphatidic acid or LPA). Third, the enzyme 1-acyl-sn-glycerol-3-phosphate acyltransferase converts LPA into phosphatidic acid (PA or 1,2-diacyl-sn-glycerol 3-phosphate) by esterifying an acyl-group to the sn-2 position of the glycerol backbone. PA is then transferred to the inner mitochondrial membrane to continue cardiolipin synthesis. Fourth, magnesium-dependent phosphatidate cytidylyltransferase catalyzes the conversion of PA into CDP-diacylglycerol. Fifth, CDP-diacylglycerol--glycerol-3-phosphate 3-phosphatidyltransferase synthesizes phosphatidylglycerophosphate (PGP). Sixth, phosphatidylglycerophosphatase and protein-tyrosine phosphatase dephosphorylates PGP to form phosphatidylglycerol (PG). Last, cardiolipin synthase catalyzes the synthesis of cardiolipin by transferring a phosphatidyl group from a second CDP-diacylglycerol to PG. It requires a divalent metal cation cofactor.
|
Metabolite
Metabolic
|
|
|
SMP0388994![]() |
Palmitate BiosynthesisBacteroides sp. 3_1_40A
Palmitate is synthesized by stepwise condensation of C2 units to a growing acyl chain. Each elongation cycle results in the addition of two carbons to the acyl chain, and consists of four separate reactions.
The pathway starts with acetyl-CoA interacting with hydrogen carbonate through an ATP driven acetyl-CoA carboxylase resulting in a phosphate, an ADP , a hydrogen ion and a malonyl-CoA. The latter compound interacts with a holo-[acp] through a malonyl-CoA-ACP transacylase resulting in a CoA and a malonyl-[acp]. This compound interacts with hydrogen ion, acetyl-CoA through a KASIII resulting in a CoA, carbon dioxide and an acetoacetyl-[acp].
The latter compound interacts with a hydrogen ion through a NADPH driven 3-oxoacyl-[acyl-carrier-protein] reductase resulting in an NADP and a (R) 3-Hydroxybutanoyl-[acp](1).
This compound is then dehydrated by a 3-hydroxyacyl-[acyl-carrier-protein] dehydratase resulting in the release of water and a crotonyl-[acp](2).
The crotonyl-[acp] interacts with a hydrogen ion through a NADH enoyl-[acyl-carrier-protein] reductase(NAD) resulting in NAD and a butyryl-[acp](3).
The butyryl-[acp] interacts with a hydrogen ion, a malonyl-[acp] through a KASI resulting in a holo-[acp],carbon dioxide and a 3-oxo-hexanoyl-[acp](4).
The 3-oxo-hexanoyl-[acp] interacts with a hydrogen ion through a NADPH driven 3-oxoacyl-[acyl-carrier-protein] reductase resulting in an NADP and a (R) 3-Hydroxyhexanoyl-[acp](1).
This compound is then dehydrated by a 3-hydroxyacyl-[acyl-carrier-protein] dehydratase resulting in the release of water and a trans hex-2-enoyl-[acp](2).
The trans hex-2-enoyl-[acp] interacts with a hydrogen ion through a NADH enoyl-[acyl-carrier-protein] reductase(NAD) resulting in NAD and a hexanoyl-[acp](3).
The hexanoyl-[acp] interacts with a hydrogen ion, a malonyl-[acp] through a KASI resulting in a holo-[acp],carbon dioxide and a 3-oxo-octanoyl-[acp](4).
The 3-oxo-octanoyl-[acp] interacts with a hydrogen ion through a NADPH driven 3-oxoacyl-[acyl-carrier-protein] reductase resulting in an NADP and a (R) 3-Hydroxyoctanoyl-[acp](1).
This compound is then dehydrated by a 3-hydroxyacyl-[acyl-carrier-protein] dehydratase resulting in the release of water and a trans oct-2-enoyl-[acp](2).
The trans oct-2-enoyl-[acp] interacts with a hydrogen ion through a NADH enoyl-[acyl-carrier-protein] reductase(NAD) resulting in NAD and a octanoyl-[acp](3).
The octanoyl-[acp] interacts with a hydrogen ion, a malonyl-[acp] through a KASI resulting in a holo-[acp],carbon dioxide and a 3-oxo-decanoyl-[acp](4).
The 3-oxo-decanoyl-[acp] interacts with a hydrogen ion through a NADPH driven 3-oxoacyl-[acyl-carrier-protein] reductase resulting in an NADP and a (R) 3-Hydroxydecanoyl-[acp](1).
This compound is then dehydrated by a 3-hydroxyacyl-[acyl-carrier-protein] dehydratase resulting in the release of water and a trans-delta2-decenoyl-[acp](2).
The a trans-delta2-decenoyl-[acp] interacts with a hydrogen ion through a NADH enoyl-[acyl-carrier-protein] reductase(NAD) resulting in NAD and a decanoyl-[acp](3).
The decanoyl-[acp] interacts with a malonyl-[acp] through a KASI resulting in a holo-[acp],carbon dioxide and a 3-oxo-dodecanoyl-[acp](4).
The 3-oxo-dodecanoyl-[acp ]interacts with a hydrogen ion through a NADPH driven 3-oxoacyl-[acyl-carrier-protein] reductase resulting in an NADP and a (R) 3-Hydroxydodecanoyl-[acp](1).
This compound is then dehydrated by a 3-hydroxyacyl-[acyl-carrier-protein] dehydratase resulting in the release of water and a trans dodec-2-enoyl-[acp](2).
The trans dodec-2-enoyl-[acp] interacts with a hydrogen ion through a NADH enoyl-[acyl-carrier-protein] reductase(NAD) resulting in NAD and a dodecanoyl-[acp](3). This compound can either react with water spontaneously resulting in a hydrogen ion, a holo-[acp] and a dodecanoic acid or it interacts with a hydrogen ion, a malonyl-[acp] through a KASI resulting in a holo-[acp],carbon dioxide and a 3-oxo-myristoyl-[acp](4).
The 3-oxo-myristoyl-[acp] interacts with a hydrogen ion through a NADPH driven 3-oxoacyl-[acyl-carrier-protein] reductase resulting in an NADP and a (3R) 3-Hydroxymyristoyl-[acp](1).
This compound is then dehydrated by a 3-hydroxyacyl-[acyl-carrier-protein] dehydratase resulting in the release of water and a trans tetradec-2-enoyl-[acp](2).
This compound interacts with a hydrogen ion, through a NADH-driven KASI resulting in a NAD and a myristoyl-[acp].
Myristoyl-[acp] with a hydrogen ion, a malonyl-[acp] through a KASI resulting in a holo-[acp],carbon dioxide and a 3-oxo-palmitoyl-[acp](4).
The 3-oxo-palmitoyl-[acp] interacts with a hydrogen ion through a NADPH driven 3-oxoacyl-[acyl-carrier-protein] reductase resulting in an NADP and a (3R) 3-Hydroxypalmitoyl-[acp](1).
This compound is then dehydrated by a 3-hydroxyacyl-[acyl-carrier-protein] dehydratase resulting in the release of water and a trans hexadecenoyl-[acp](2).
The trans hexadecenoyl-[acp] interacts with a hydrogen ion through a NADH enoyl-[acyl-carrier-protein] reductase(NAD) resulting in NAD and a palmitoyl-[acp](3).
Palmitoyl then reacts with water spontaneously resulting in a hydrogen ion, a holo-[acp] and palmitic acid.
No integral membrane protein required for long chain fatty acid uptake has been identified in E. coli. The transport of long chain fatty acids across the cytoplasmic membrane is dependent on fatty acyl-CoA synthetase. An energised membrane is necessary for fatty acid transport and it has been suggested that uncharged fatty acids flip across the inner membrane by diffusion.
|
Metabolite
Metabolic
|
||
SMP0399252![]() |
Gluconeogenesis from L-Malic AcidCampylobacter gracilis RM3268
Gluconeogenesis from L-malic acid starts from the introduction of L-malic acid into cytoplasm either through a C4 dicarboxylate / orotate:H+ symporter or a dicarboxylate transporter (succinic acid antiporter). L-malic acid is then metabolized through 3 possible ways: NAD driven malate dehydrogenase resulting in oxalacetic acid, NADP driven malate dehydrogenase B resulting pyruvic acid or malate dehydrogenase, NAD-requiring resulting in pyruvic acid.
Oxalacetic acid is processed by phosphoenolpyruvate carboxykinase (ATP driven) while pyruvic acid is processed by phosphoenolpyruvate synthetase resulting in phosphoenolpyruvic acid. This compound is dehydrated by enolase resulting in an 2-phosphoglyceric acid which is then isomerized by 2,3-bisphosphoglycerate-independent phosphoglycerate mutase resulting in a 3-phosphoglyceric acid which is phosphorylated by an ATP driven phosphoglycerate kinase resulting in a glyceric acid 1,3-biphosphate. This compound undergoes an NADH driven glyceraldehyde 3-phosphate dehydrogenase reaction resulting in a D-Glyceraldehyde 3-phosphate which is first isomerized into dihydroxyacetone phosphate through an triosephosphate isomerase. D-glyceraldehyde 3-phosphate and Dihydroxyacetone phosphate react through a fructose biphosphate aldolase protein complex resulting in a fructose 1,6-biphosphate. Fructose 1,6-biphosphateis is metabolized by a fructose-1,6-bisphosphatase resulting in a Beta-D-fructofuranose 6-phosphate which is then isomerized into a Beta-D-glucose 6-phosphate through a glucose-6-phosphate isomerase.
|
Metabolite
Metabolic
|
|
|
Showing 348431 -
348440 of 351079 pathways