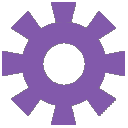
Browsing Pathways
Showing 348891 -
348900 of 605359 pathways
PathBank ID | Pathway Name and Description | Pathway Class | Chemical Compounds | Proteins |
---|---|---|---|---|
SMP0390700![]() |
Tyrosine BiosynthesisBacteroides intestinalis
Tyrosine is one of the amino acid used in protein synthesis. The tyrosine biosynthesis pathways is connected with the chorismate biosynthesis pathway. Chorismate biosynthesis produce the chorismate, which can further be converted to prephenate by T-protein. Combined with cofactor, NAD, prephenate has been further converted to 4-Hydroxyphenylpyruvic acid by T-protein with generated NADH and carbon dioxide. Tyrosine aminotransferase catalyzes 4-Hydroxyphenylpyruvic acid to tyrosine, and also converts glutamic acid to oxoglutaric acid. Tyrosine will be further catalyzed into various molecules such as 2-iminoacetate, p-Cresol, 5'Deoxyadenosine and L-Methionine; or it will be exported from cell via the lysine exporter.
|
Metabolite
Metabolic
|
||
SMP0322139 |
Serine Biosynthesis and MetabolismBacteroides intestinalis
Serine biosynthesis is a major metabolic pathway in E. coli. Its end product, serine, is not only used in protein synthesis, but also as a precursor for the biosynthesis of glycine, cysteine, tryptophan, and phospholipids. In addition, it directly or indirectly serves as a source of one-carbon units for the biosynthesis of various compounds.
The biosynthesis of serine starts with 3-phosphoglyceric acid being metabolized by a NAD driven D-3-phosphoglycerate dehydrogenase / α-ketoglutarate reductase resulting in the release of a NADH, a hydrogen ion and a phosphohydroxypyruvic acid. The latter compound then interacts with an L-glutamic acid through a 3-phosphoserine aminotransferase / phosphohydroxythreonine aminotransferase resulting in oxoglutaric acid and DL-D-phosphoserine.
The DL-D-phosphoserine can also be imported into the cytoplasm through a phosphonate ABC transporter. The DL-D-phosphoserine is dephosphorylated by interacting with a water molecule through a phosphoserine phosphatase resulting in the release of a phosphate and an L-serine
L-serine is then metabolized by being dehydrated through either a L-serine dehydratase 2 or a L-serine dehydratase 1 resulting in the release of a water molecule, a hydrogen ion and a 2-aminoacrylic acid. The latter compound is an isomer of a 2-iminopropanoate which reacts spontaneously with a water molecule and a hydrogen ion resulting in the release of Ammonium and pyruvic acid. Pyruvic acid then interacts with a coenzyme A through a NAD driven pyruvate dehydrogenase complex resulting in the release of a NADH, a carbon dioxide and an acetyl-CoA.
|
Metabolite
Metabolic
|
|
|
SMP0292183 |
Cardiolipin Biosynthesis CL(18:1(9Z)/18:2(9Z,11Z)/18:1(9Z)/16:0)Mus musculus
Cardiolipin (CL) is an important component of the inner mitochondrial membrane where it constitutes about 20% of the total lipid composition. It is essential for the optimal function of numerous enzymes that are involved in mitochondrial energy metabolism (Wikipedia). Cardiolipin biosynthesis occurs mainly in the mitochondria, but there also exists an alternative synthesis route for CDP-diacylglycerol that takes place in the endoplasmic reticulum. This second route may supplement this pathway. All membrane-localized enzymes are coloured dark green in the image. First, dihydroxyacetone phosphate (or glycerone phosphate) from glycolysis is used by the cytosolic enzyme glycerol-3-phosphate dehydrogenase [NAD(+)] to synthesize sn-glycerol 3-phosphate. Second, the mitochondrial outer membrane enzyme glycerol-3-phosphate acyltransferase esterifies an acyl-group to the sn-1 position of sn-glycerol 3-phosphate to form 1-acyl-sn-glycerol 3-phosphate (lysophosphatidic acid or LPA). Third, the enzyme 1-acyl-sn-glycerol-3-phosphate acyltransferase converts LPA into phosphatidic acid (PA or 1,2-diacyl-sn-glycerol 3-phosphate) by esterifying an acyl-group to the sn-2 position of the glycerol backbone. PA is then transferred to the inner mitochondrial membrane to continue cardiolipin synthesis. Fourth, magnesium-dependent phosphatidate cytidylyltransferase catalyzes the conversion of PA into CDP-diacylglycerol. Fifth, CDP-diacylglycerol--glycerol-3-phosphate 3-phosphatidyltransferase synthesizes phosphatidylglycerophosphate (PGP). Sixth, phosphatidylglycerophosphatase and protein-tyrosine phosphatase dephosphorylates PGP to form phosphatidylglycerol (PG). Last, cardiolipin synthase catalyzes the synthesis of cardiolipin by transferring a phosphatidyl group from a second CDP-diacylglycerol to PG. It requires a divalent metal cation cofactor.
|
Metabolite
Metabolic
|
|
|
SMP0397341![]() |
Tyrosine BiosynthesisBacteroides coprocola
Tyrosine is one of the amino acid used in protein synthesis. The tyrosine biosynthesis pathways is connected with the chorismate biosynthesis pathway. Chorismate biosynthesis produce the chorismate, which can further be converted to prephenate by T-protein. Combined with cofactor, NAD, prephenate has been further converted to 4-Hydroxyphenylpyruvic acid by T-protein with generated NADH and carbon dioxide. Tyrosine aminotransferase catalyzes 4-Hydroxyphenylpyruvic acid to tyrosine, and also converts glutamic acid to oxoglutaric acid. Tyrosine will be further catalyzed into various molecules such as 2-iminoacetate, p-Cresol, 5'Deoxyadenosine and L-Methionine; or it will be exported from cell via the lysine exporter.
|
Metabolite
Metabolic
|
||
SMP0390707![]() |
Glycine BiosynthesisBacteroides intestinalis
Glycine biosynthesis is dependent on L-serine. L-serine is enters the cell through transporters (serine / threonine:H+ symporter TdcC, serine/threonine: Na symporter , serine:H+ symporter SdaC) and then proceeds through reversible reaction with a tetrahydrofolic acid through a serine hydroxymethyltransferase enzyme in order to produce glycine, 5,10-methylene tetrahydrofolate and water. 5,10-methylene tetrahydrofolate is a major source of one-carbon units used in other metabolic pathways.
|
Metabolite
Metabolic
|
||
SMP0292247 |
Cardiolipin Biosynthesis CL(18:1(9Z)/18:2(9Z,12Z)/16:1(9Z)/20:5(5Z,8Z,11Z,14Z,17Z))Mus musculus
Cardiolipin (CL) is an important component of the inner mitochondrial membrane where it constitutes about 20% of the total lipid composition. It is essential for the optimal function of numerous enzymes that are involved in mitochondrial energy metabolism (Wikipedia). Cardiolipin biosynthesis occurs mainly in the mitochondria, but there also exists an alternative synthesis route for CDP-diacylglycerol that takes place in the endoplasmic reticulum. This second route may supplement this pathway. All membrane-localized enzymes are coloured dark green in the image. First, dihydroxyacetone phosphate (or glycerone phosphate) from glycolysis is used by the cytosolic enzyme glycerol-3-phosphate dehydrogenase [NAD(+)] to synthesize sn-glycerol 3-phosphate. Second, the mitochondrial outer membrane enzyme glycerol-3-phosphate acyltransferase esterifies an acyl-group to the sn-1 position of sn-glycerol 3-phosphate to form 1-acyl-sn-glycerol 3-phosphate (lysophosphatidic acid or LPA). Third, the enzyme 1-acyl-sn-glycerol-3-phosphate acyltransferase converts LPA into phosphatidic acid (PA or 1,2-diacyl-sn-glycerol 3-phosphate) by esterifying an acyl-group to the sn-2 position of the glycerol backbone. PA is then transferred to the inner mitochondrial membrane to continue cardiolipin synthesis. Fourth, magnesium-dependent phosphatidate cytidylyltransferase catalyzes the conversion of PA into CDP-diacylglycerol. Fifth, CDP-diacylglycerol--glycerol-3-phosphate 3-phosphatidyltransferase synthesizes phosphatidylglycerophosphate (PGP). Sixth, phosphatidylglycerophosphatase and protein-tyrosine phosphatase dephosphorylates PGP to form phosphatidylglycerol (PG). Last, cardiolipin synthase catalyzes the synthesis of cardiolipin by transferring a phosphatidyl group from a second CDP-diacylglycerol to PG. It requires a divalent metal cation cofactor.
|
Metabolite
Metabolic
|
|
|
SMP0292252 |
Cardiolipin Biosynthesis CL(18:1(9Z)/18:2(9Z,12Z)/18:0/16:0)Mus musculus
Cardiolipin (CL) is an important component of the inner mitochondrial membrane where it constitutes about 20% of the total lipid composition. It is essential for the optimal function of numerous enzymes that are involved in mitochondrial energy metabolism (Wikipedia). Cardiolipin biosynthesis occurs mainly in the mitochondria, but there also exists an alternative synthesis route for CDP-diacylglycerol that takes place in the endoplasmic reticulum. This second route may supplement this pathway. All membrane-localized enzymes are coloured dark green in the image. First, dihydroxyacetone phosphate (or glycerone phosphate) from glycolysis is used by the cytosolic enzyme glycerol-3-phosphate dehydrogenase [NAD(+)] to synthesize sn-glycerol 3-phosphate. Second, the mitochondrial outer membrane enzyme glycerol-3-phosphate acyltransferase esterifies an acyl-group to the sn-1 position of sn-glycerol 3-phosphate to form 1-acyl-sn-glycerol 3-phosphate (lysophosphatidic acid or LPA). Third, the enzyme 1-acyl-sn-glycerol-3-phosphate acyltransferase converts LPA into phosphatidic acid (PA or 1,2-diacyl-sn-glycerol 3-phosphate) by esterifying an acyl-group to the sn-2 position of the glycerol backbone. PA is then transferred to the inner mitochondrial membrane to continue cardiolipin synthesis. Fourth, magnesium-dependent phosphatidate cytidylyltransferase catalyzes the conversion of PA into CDP-diacylglycerol. Fifth, CDP-diacylglycerol--glycerol-3-phosphate 3-phosphatidyltransferase synthesizes phosphatidylglycerophosphate (PGP). Sixth, phosphatidylglycerophosphatase and protein-tyrosine phosphatase dephosphorylates PGP to form phosphatidylglycerol (PG). Last, cardiolipin synthase catalyzes the synthesis of cardiolipin by transferring a phosphatidyl group from a second CDP-diacylglycerol to PG. It requires a divalent metal cation cofactor.
|
Metabolite
Metabolic
|
|
|
SMP0292353 |
Cardiolipin Biosynthesis CL(18:1(9Z)/18:2(9Z,12Z)/20:5(5Z,8Z,11Z,14Z,17Z)/22:5(7Z,10Z,13Z,16Z,19Z))Mus musculus
Cardiolipin (CL) is an important component of the inner mitochondrial membrane where it constitutes about 20% of the total lipid composition. It is essential for the optimal function of numerous enzymes that are involved in mitochondrial energy metabolism (Wikipedia). Cardiolipin biosynthesis occurs mainly in the mitochondria, but there also exists an alternative synthesis route for CDP-diacylglycerol that takes place in the endoplasmic reticulum. This second route may supplement this pathway. All membrane-localized enzymes are coloured dark green in the image. First, dihydroxyacetone phosphate (or glycerone phosphate) from glycolysis is used by the cytosolic enzyme glycerol-3-phosphate dehydrogenase [NAD(+)] to synthesize sn-glycerol 3-phosphate. Second, the mitochondrial outer membrane enzyme glycerol-3-phosphate acyltransferase esterifies an acyl-group to the sn-1 position of sn-glycerol 3-phosphate to form 1-acyl-sn-glycerol 3-phosphate (lysophosphatidic acid or LPA). Third, the enzyme 1-acyl-sn-glycerol-3-phosphate acyltransferase converts LPA into phosphatidic acid (PA or 1,2-diacyl-sn-glycerol 3-phosphate) by esterifying an acyl-group to the sn-2 position of the glycerol backbone. PA is then transferred to the inner mitochondrial membrane to continue cardiolipin synthesis. Fourth, magnesium-dependent phosphatidate cytidylyltransferase catalyzes the conversion of PA into CDP-diacylglycerol. Fifth, CDP-diacylglycerol--glycerol-3-phosphate 3-phosphatidyltransferase synthesizes phosphatidylglycerophosphate (PGP). Sixth, phosphatidylglycerophosphatase and protein-tyrosine phosphatase dephosphorylates PGP to form phosphatidylglycerol (PG). Last, cardiolipin synthase catalyzes the synthesis of cardiolipin by transferring a phosphatidyl group from a second CDP-diacylglycerol to PG. It requires a divalent metal cation cofactor.
|
Metabolite
Metabolic
|
|
|
SMP0292355 |
Cardiolipin Biosynthesis CL(18:1(9Z)/18:2(9Z,12Z)/22:5(4Z,7Z,10Z,13Z,16Z)/14:0)Mus musculus
Cardiolipin (CL) is an important component of the inner mitochondrial membrane where it constitutes about 20% of the total lipid composition. It is essential for the optimal function of numerous enzymes that are involved in mitochondrial energy metabolism (Wikipedia). Cardiolipin biosynthesis occurs mainly in the mitochondria, but there also exists an alternative synthesis route for CDP-diacylglycerol that takes place in the endoplasmic reticulum. This second route may supplement this pathway. All membrane-localized enzymes are coloured dark green in the image. First, dihydroxyacetone phosphate (or glycerone phosphate) from glycolysis is used by the cytosolic enzyme glycerol-3-phosphate dehydrogenase [NAD(+)] to synthesize sn-glycerol 3-phosphate. Second, the mitochondrial outer membrane enzyme glycerol-3-phosphate acyltransferase esterifies an acyl-group to the sn-1 position of sn-glycerol 3-phosphate to form 1-acyl-sn-glycerol 3-phosphate (lysophosphatidic acid or LPA). Third, the enzyme 1-acyl-sn-glycerol-3-phosphate acyltransferase converts LPA into phosphatidic acid (PA or 1,2-diacyl-sn-glycerol 3-phosphate) by esterifying an acyl-group to the sn-2 position of the glycerol backbone. PA is then transferred to the inner mitochondrial membrane to continue cardiolipin synthesis. Fourth, magnesium-dependent phosphatidate cytidylyltransferase catalyzes the conversion of PA into CDP-diacylglycerol. Fifth, CDP-diacylglycerol--glycerol-3-phosphate 3-phosphatidyltransferase synthesizes phosphatidylglycerophosphate (PGP). Sixth, phosphatidylglycerophosphatase and protein-tyrosine phosphatase dephosphorylates PGP to form phosphatidylglycerol (PG). Last, cardiolipin synthase catalyzes the synthesis of cardiolipin by transferring a phosphatidyl group from a second CDP-diacylglycerol to PG. It requires a divalent metal cation cofactor.
|
Metabolite
Metabolic
|
|
|
SMP0397987![]() |
Palmitate BiosynthesisEscherichia coli str. K-12 substr. DH10B
Palmitate is synthesized by stepwise condensation of C2 units to a growing acyl chain. Each elongation cycle results in the addition of two carbons to the acyl chain, and consists of four separate reactions.
The pathway starts with acetyl-CoA interacting with hydrogen carbonate through an ATP driven acetyl-CoA carboxylase resulting in a phosphate, an ADP , a hydrogen ion and a malonyl-CoA. The latter compound interacts with a holo-[acp] through a malonyl-CoA-ACP transacylase resulting in a CoA and a malonyl-[acp]. This compound interacts with hydrogen ion, acetyl-CoA through a KASIII resulting in a CoA, carbon dioxide and an acetoacetyl-[acp].
The latter compound interacts with a hydrogen ion through a NADPH driven 3-oxoacyl-[acyl-carrier-protein] reductase resulting in an NADP and a (R) 3-Hydroxybutanoyl-[acp](1).
This compound is then dehydrated by a 3-hydroxyacyl-[acyl-carrier-protein] dehydratase resulting in the release of water and a crotonyl-[acp](2).
The crotonyl-[acp] interacts with a hydrogen ion through a NADH enoyl-[acyl-carrier-protein] reductase(NAD) resulting in NAD and a butyryl-[acp](3).
The butyryl-[acp] interacts with a hydrogen ion, a malonyl-[acp] through a KASI resulting in a holo-[acp],carbon dioxide and a 3-oxo-hexanoyl-[acp](4).
The 3-oxo-hexanoyl-[acp] interacts with a hydrogen ion through a NADPH driven 3-oxoacyl-[acyl-carrier-protein] reductase resulting in an NADP and a (R) 3-Hydroxyhexanoyl-[acp](1).
This compound is then dehydrated by a 3-hydroxyacyl-[acyl-carrier-protein] dehydratase resulting in the release of water and a trans hex-2-enoyl-[acp](2).
The trans hex-2-enoyl-[acp] interacts with a hydrogen ion through a NADH enoyl-[acyl-carrier-protein] reductase(NAD) resulting in NAD and a hexanoyl-[acp](3).
The hexanoyl-[acp] interacts with a hydrogen ion, a malonyl-[acp] through a KASI resulting in a holo-[acp],carbon dioxide and a 3-oxo-octanoyl-[acp](4).
The 3-oxo-octanoyl-[acp] interacts with a hydrogen ion through a NADPH driven 3-oxoacyl-[acyl-carrier-protein] reductase resulting in an NADP and a (R) 3-Hydroxyoctanoyl-[acp](1).
This compound is then dehydrated by a 3-hydroxyacyl-[acyl-carrier-protein] dehydratase resulting in the release of water and a trans oct-2-enoyl-[acp](2).
The trans oct-2-enoyl-[acp] interacts with a hydrogen ion through a NADH enoyl-[acyl-carrier-protein] reductase(NAD) resulting in NAD and a octanoyl-[acp](3).
The octanoyl-[acp] interacts with a hydrogen ion, a malonyl-[acp] through a KASI resulting in a holo-[acp],carbon dioxide and a 3-oxo-decanoyl-[acp](4).
The 3-oxo-decanoyl-[acp] interacts with a hydrogen ion through a NADPH driven 3-oxoacyl-[acyl-carrier-protein] reductase resulting in an NADP and a (R) 3-Hydroxydecanoyl-[acp](1).
This compound is then dehydrated by a 3-hydroxyacyl-[acyl-carrier-protein] dehydratase resulting in the release of water and a trans-delta2-decenoyl-[acp](2).
The a trans-delta2-decenoyl-[acp] interacts with a hydrogen ion through a NADH enoyl-[acyl-carrier-protein] reductase(NAD) resulting in NAD and a decanoyl-[acp](3).
The decanoyl-[acp] interacts with a malonyl-[acp] through a KASI resulting in a holo-[acp],carbon dioxide and a 3-oxo-dodecanoyl-[acp](4).
The 3-oxo-dodecanoyl-[acp ]interacts with a hydrogen ion through a NADPH driven 3-oxoacyl-[acyl-carrier-protein] reductase resulting in an NADP and a (R) 3-Hydroxydodecanoyl-[acp](1).
This compound is then dehydrated by a 3-hydroxyacyl-[acyl-carrier-protein] dehydratase resulting in the release of water and a trans dodec-2-enoyl-[acp](2).
The trans dodec-2-enoyl-[acp] interacts with a hydrogen ion through a NADH enoyl-[acyl-carrier-protein] reductase(NAD) resulting in NAD and a dodecanoyl-[acp](3). This compound can either react with water spontaneously resulting in a hydrogen ion, a holo-[acp] and a dodecanoic acid or it interacts with a hydrogen ion, a malonyl-[acp] through a KASI resulting in a holo-[acp],carbon dioxide and a 3-oxo-myristoyl-[acp](4).
The 3-oxo-myristoyl-[acp] interacts with a hydrogen ion through a NADPH driven 3-oxoacyl-[acyl-carrier-protein] reductase resulting in an NADP and a (3R) 3-Hydroxymyristoyl-[acp](1).
This compound is then dehydrated by a 3-hydroxyacyl-[acyl-carrier-protein] dehydratase resulting in the release of water and a trans tetradec-2-enoyl-[acp](2).
This compound interacts with a hydrogen ion, through a NADH-driven KASI resulting in a NAD and a myristoyl-[acp].
Myristoyl-[acp] with a hydrogen ion, a malonyl-[acp] through a KASI resulting in a holo-[acp],carbon dioxide and a 3-oxo-palmitoyl-[acp](4).
The 3-oxo-palmitoyl-[acp] interacts with a hydrogen ion through a NADPH driven 3-oxoacyl-[acyl-carrier-protein] reductase resulting in an NADP and a (3R) 3-Hydroxypalmitoyl-[acp](1).
This compound is then dehydrated by a 3-hydroxyacyl-[acyl-carrier-protein] dehydratase resulting in the release of water and a trans hexadecenoyl-[acp](2).
The trans hexadecenoyl-[acp] interacts with a hydrogen ion through a NADH enoyl-[acyl-carrier-protein] reductase(NAD) resulting in NAD and a palmitoyl-[acp](3).
Palmitoyl then reacts with water spontaneously resulting in a hydrogen ion, a holo-[acp] and palmitic acid.
No integral membrane protein required for long chain fatty acid uptake has been identified in E. coli. The transport of long chain fatty acids across the cytoplasmic membrane is dependent on fatty acyl-CoA synthetase. An energised membrane is necessary for fatty acid transport and it has been suggested that uncharged fatty acids flip across the inner membrane by diffusion.
|
Metabolite
Metabolic
|
|
Showing 348891 -
348900 of 350957 pathways