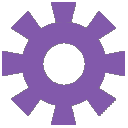
Browsing Pathways
Showing 349341 -
349350 of 605359 pathways
PathBank ID | Pathway Name and Description | Pathway Class | Chemical Compounds | Proteins |
---|---|---|---|---|
SMP0402013![]() |
Fucose and Rhamnose DegradationRoseburia hominis A2-183
In E. coli, L-fucose and L-rhamnose are metabolized through parallel pathways. The pathways converge after their corresponding aldolase reactions yielding the same products: lactaldehye. Proton symporter can facilitate the import of alpha-L-rhamnopyranose, methylpentose and beta-L-rhamnopyranose into cell for further metabolism, which allow E.coli to grow with carbon and energy. For alpha-L-rhamnopyranose, it is isomerized by a l-rhamnose mutarotase resulting in a beta-L-rhamnopyranose which is then isomerized into a keto-L-rhamnulose by a l-rhamnose isomerase. The keto-L-rhamnulose spontaneously changes into a L-rhamnulofuranose which is phosphorylated by a rhamnulokinase resulting in a L-rhamnulose 1-phosphate. This compound reacts with a rhamnulose-1-phosphate aldolase resulting in a dihydroxyacetone phosphate and a lactaldehyde. For beta-L-rhamnopyranose, it is isomerized by a L-fucose mutarotase resulting in a alpha-L-fucopyranose. This compound is then isomerized by an L-fucose isomerase resulting in a L-fuculose which in turn gets phosphorylated into an L-fuculose 1-phosphate through an L-fuculokinase. The compound L-fuculose 1-phosphate reacts with an L-fuculose phosphate aldolase through a dihydroxyacetone phosphate and a lactaldehyde. Two pathways can both be used for degrading L-lactaldehyde, which the aerobic pathway facilitates the conversion from L-lactic acid to pyruvic acid via L-lactate dehydrogenase, and the anaerobic pathway facilitates conversion from lactaldehyde to propane-1,2-diol via lactaldehyde reductase. Under aerobic conditions, L-lactaldehyde is oxidized in two steps to pyruvate, thereby channeling all the carbons from fucose or rhamnose into central metabolic pathways. Under anaerobic conditions, L-lactaldehyde is reduced to L-1,2-propanediol, which is secreted into the environment.
|
Metabolite
Metabolic
|
|
|
SMP0402018![]() |
Glycolate and Glyoxylate DegradationPrevotella ruminicola 23
Glycolic acid is introduced into the cytoplasm through either a glycolate / lactate:H+ symporter or a acetate / glycolate transporter. Once inside, glycolic acid reacts with an oxidized electron-transfer flavoprotein through a glycolate oxidase resulting in a reduced acceptor and glyoxylic acid. Glyoxylic acid can also be obtained from the introduction of glyoxylic acid. It can also be obtained from the metabolism of (S)-allantoin.
S-allantoin is introduced into the cytoplasm through a purine and pyrimidine transporter(allantoin specific). Once inside, the compound reacts with water through a allantoinase resulting in hydrogen ion and allantoic acid. Allantoic acid then reacts with water and hydrogen ion through a allantoate amidohydrolase resulting in a carbon dioxide, ammonium and S-ureidoglycine. The latter compound reacts with water through a S-ureidoglycine aminohydrolase resulting in ammonium and S-ureidoglycolic acid which in turn reacts with a Ureidoglycolate lyase resulting in urea and glyoxylic acid.
Glyoxylic acid can either be metabolized into L-malic acid by a reaction with acetyl-CoA and Water through a malate synthase G which also releases hydrogen ion and Coenzyme A. L-malic acid is then incorporated into the TCA cycle.
Glyoxylic acid can also be metabolized by glyoxylate carboligase, releasing a carbon dioxide and tartronate semialdehyde. The latter compound is then reduced by an NADH driven tartronate semialdehyde reductase 2 resulting in glyceric acid. Glyceric acid is phosphorylated by a glycerate kinase 2 resulting in a 3-phosphoglyceric acid. This compound is then integrated into various other pathways: cysteine biosynthesis, serine biosynthesis and glycolysis and pyruvate dehydrogenase.
|
Metabolite
Metabolic
|
|
|
SMP0402003![]() |
Galactitol and Galactonate DegradationRoseburia hominis A2-183
Escherichia coli can solely use D-galactonate as a carbon and energy source. The initial step, after the transport of galactonic acid into the cell is the dehydration of D-galactonate to 2-dehydro-3-deoxy-D-galactonate by D-galactonate dehydratase. Subsequent phosphorylation by 2-dehydro-3-deoxygalactonate kinase and aldol cleavage by 2-oxo-3-deoxygalactonate 6-phosphate aldolase produces pyruvate and D-glyceraldehyde-3-phosphate, which enter central metabolism. Galactitol can also be utilized by E. coli K-12 as the sole source of carbon and energy. Each enters the cell via a specific phosphotransferase system, so the first intracellular species is D-galactitol-1-phosphate or D-galactitol-6-phosphate, which are identical. This sugar alcohol phosphate becomes the substrate for a dehydrogenase that oxidizes its 2-alcohol group to a keto group. Galactitol-1-phosphate is dehydrogenated to tagatose-6-phosphate which is then acted on by a kinase and an aldose and eventually is converted to glycolysis intermediates.
|
Metabolite
Metabolic
|
|
|
SMP0402009![]() |
Mannose MetabolismVeillonella parvula DSM 2008
Escherichia coli can utilize D-mannose for its sole carbon and energy source. Alpha-D-mannose is introduced into the cytoplasm through a mannose PTS permease. A phosphotransferase system (PTS) takes up mannose producing D-mannose-6-phosphate which is then converted to D-fructose-6-phosphate via an isomerase. D-fructose-6-phosphate is an intermediate of glycolysis and can enter the pathways of metabolism. The first two enzymes in the pathway catalyze isomerizations that interconvert phosphorylated aldohexoses (β-D-glucose-6-phosphate, D-mannose-6-phosphate) and phosphorylated ketohexoses (D-fructose-6-phosphate). The reaction catalyzed by mannose-6-phosphate isomerase that produces D-mannose-6-phosphate is the first committed step in the biosynthesis of the activated mannose donor GDP-α-D-mannose. D-mannose-6-phosphate is then converted to GDP-D-mannose by the interaction of phosphomannomutase and mannose-1-phosphate guanylyltransferase. GDP-D-mannose produces GDP-L-fucose beginning with the dehydration to GDP-4-dehydro-6-deoxy-D-mannose. GDP-fucose is synthesized by a two step epimerase and reductase of GDP-4-dehydro-6-deoxy-D-mannose. L-fucose then enters the colanic acid building blocks biosynthesis pathway.
|
Metabolite
Metabolic
|
||
SMP0402068![]() |
Glutathione MetabolismMethylococcus capsulatus
The biosynthesis of glutathione starts with the introduction of L-glutamic acid through either a glutamate:sodium symporter, glutamate / aspartate : H+ symporter GltP or a
glutamate / aspartate ABC transporter. Once in the cytoplasm, L-glutamice acid reacts with L-cysteine through an ATP glutamate-cysteine ligase resulting in gamma-glutamylcysteine. This compound reacts which Glycine through an ATP driven glutathione synthetase thus catabolizing Glutathione.
This compound is metabolized through a spontaneous reaction with an oxidized glutaredoxin resulting in a reduced glutaredoxin and an oxidized glutathione. This compound is reduced by a NADPH glutathione reductase resulting in a glutathione.
Glutathione can then be degraded into various different glutathione containing compounds by reacting with a napthalene or Bromobenzene-2,3-oxide through a glutathione S-transferase.
|
Metabolite
Metabolic
|
|
|
SMP0402072![]() |
Galactose MetabolismBacteroides coprocola
Galactose can be synthesized through two pathways: melibiose degradation involving an alpha galactosidase and lactose degradation involving a beta galactosidase. Melibiose is first transported inside the cell through the melibiose:Li+/Na+/H+ symporter. Once inside the cell, melibiose is degraded through alpha galactosidase into an alpha-D-galactose and a beta-D-glucose. The beta-D-glucose is phosphorylated by a glucokinase to produce a beta-D-glucose-6-phosphate which can spontaneously be turned into a alpha D glucose 6 phosphate. This alpha D-glucose-6-phosphate is metabolized into a glucose -1-phosphate through a phosphoglucomutase-1. The glucose -1-phosphate is transformed into a uridine diphosphate glucose through UTP--glucose-1-phosphate uridylyltransferase. The product, uridine diphosphate glucose, can undergo a reversible reaction in which it can be turned into uridine diphosphategalactose through an UDP-glucose 4-epimerase.
Galactose can also be produced by lactose degradation involving a lactose permease to uptake lactose from the environment and a beta-galactosidase to turn lactose into Beta-D-galactose.
Beta-D-galactose can also be uptaken from the environment through a galactose proton symporter.
Galactose is degraded through the following process:
Beta-D-galactose is introduced into the cytoplasm through a galactose proton symporter, or it can be synthesized from an alpha lactose that is introduced into the cytoplasm through a lactose permease. Alpha lactose interacts with water through a beta-galactosidase resulting in a beta-D-glucose and beta-D-galactose. Beta-D-galactose is isomerized into D-galactose. D-Galactose undergoes phosphorylation through a galactokinase, hence producing galactose 1 phosphate. On the other side of the pathway, a gluose-1-phosphate (product of the interaction of alpha-D-glucose 6-phosphate with a phosphoglucomutase resulting in a alpha-D-glucose-1-phosphate, an isomer of Glucose 1-phosphate, or an isomer of Beta-D-glucose 1-phosphate) interacts with UTP and a hydrogen ion in order to produce a uridine diphosphate glucose. This is followed by the interaction of galactose-1-phosphate with an established amount of uridine diphosphate glucose through a galactose-1-phosphate uridylyltransferase, which in turn output a glucose-1-phosphate and a uridine diphosphate galactose. The glucose -1-phosphate is transformed into a uridine diphosphate glucose through UTP--glucose-1-phosphate uridylyltransferase. The product, uridine diphosphate glucose, can undergo a reversible reaction in which it can be turned into uridine diphosphategalactose through an UDP-glucose 4-epimerase, and so the cycle can keep going as long as more lactose or galactose is imported into the cell
|
Metabolite
Metabolic
|
|
|
SMP0402070![]() |
Galactose MetabolismBacteroides sp. 2_1_33B
Galactose can be synthesized through two pathways: melibiose degradation involving an alpha galactosidase and lactose degradation involving a beta galactosidase. Melibiose is first transported inside the cell through the melibiose:Li+/Na+/H+ symporter. Once inside the cell, melibiose is degraded through alpha galactosidase into an alpha-D-galactose and a beta-D-glucose. The beta-D-glucose is phosphorylated by a glucokinase to produce a beta-D-glucose-6-phosphate which can spontaneously be turned into a alpha D glucose 6 phosphate. This alpha D-glucose-6-phosphate is metabolized into a glucose -1-phosphate through a phosphoglucomutase-1. The glucose -1-phosphate is transformed into a uridine diphosphate glucose through UTP--glucose-1-phosphate uridylyltransferase. The product, uridine diphosphate glucose, can undergo a reversible reaction in which it can be turned into uridine diphosphategalactose through an UDP-glucose 4-epimerase.
Galactose can also be produced by lactose degradation involving a lactose permease to uptake lactose from the environment and a beta-galactosidase to turn lactose into Beta-D-galactose.
Beta-D-galactose can also be uptaken from the environment through a galactose proton symporter.
Galactose is degraded through the following process:
Beta-D-galactose is introduced into the cytoplasm through a galactose proton symporter, or it can be synthesized from an alpha lactose that is introduced into the cytoplasm through a lactose permease. Alpha lactose interacts with water through a beta-galactosidase resulting in a beta-D-glucose and beta-D-galactose. Beta-D-galactose is isomerized into D-galactose. D-Galactose undergoes phosphorylation through a galactokinase, hence producing galactose 1 phosphate. On the other side of the pathway, a gluose-1-phosphate (product of the interaction of alpha-D-glucose 6-phosphate with a phosphoglucomutase resulting in a alpha-D-glucose-1-phosphate, an isomer of Glucose 1-phosphate, or an isomer of Beta-D-glucose 1-phosphate) interacts with UTP and a hydrogen ion in order to produce a uridine diphosphate glucose. This is followed by the interaction of galactose-1-phosphate with an established amount of uridine diphosphate glucose through a galactose-1-phosphate uridylyltransferase, which in turn output a glucose-1-phosphate and a uridine diphosphate galactose. The glucose -1-phosphate is transformed into a uridine diphosphate glucose through UTP--glucose-1-phosphate uridylyltransferase. The product, uridine diphosphate glucose, can undergo a reversible reaction in which it can be turned into uridine diphosphategalactose through an UDP-glucose 4-epimerase, and so the cycle can keep going as long as more lactose or galactose is imported into the cell
|
Metabolite
Metabolic
|
|
|
SMP0296264 |
Cardiolipin Biosynthesis CL(18:2(9Z,12Z)/20:4(5Z,8Z,11Z,14Z)/18:1(9Z)/18:0)Mus musculus
Cardiolipin (CL) is an important component of the inner mitochondrial membrane where it constitutes about 20% of the total lipid composition. It is essential for the optimal function of numerous enzymes that are involved in mitochondrial energy metabolism (Wikipedia). Cardiolipin biosynthesis occurs mainly in the mitochondria, but there also exists an alternative synthesis route for CDP-diacylglycerol that takes place in the endoplasmic reticulum. This second route may supplement this pathway. All membrane-localized enzymes are coloured dark green in the image. First, dihydroxyacetone phosphate (or glycerone phosphate) from glycolysis is used by the cytosolic enzyme glycerol-3-phosphate dehydrogenase [NAD(+)] to synthesize sn-glycerol 3-phosphate. Second, the mitochondrial outer membrane enzyme glycerol-3-phosphate acyltransferase esterifies an acyl-group to the sn-1 position of sn-glycerol 3-phosphate to form 1-acyl-sn-glycerol 3-phosphate (lysophosphatidic acid or LPA). Third, the enzyme 1-acyl-sn-glycerol-3-phosphate acyltransferase converts LPA into phosphatidic acid (PA or 1,2-diacyl-sn-glycerol 3-phosphate) by esterifying an acyl-group to the sn-2 position of the glycerol backbone. PA is then transferred to the inner mitochondrial membrane to continue cardiolipin synthesis. Fourth, magnesium-dependent phosphatidate cytidylyltransferase catalyzes the conversion of PA into CDP-diacylglycerol. Fifth, CDP-diacylglycerol--glycerol-3-phosphate 3-phosphatidyltransferase synthesizes phosphatidylglycerophosphate (PGP). Sixth, phosphatidylglycerophosphatase and protein-tyrosine phosphatase dephosphorylates PGP to form phosphatidylglycerol (PG). Last, cardiolipin synthase catalyzes the synthesis of cardiolipin by transferring a phosphatidyl group from a second CDP-diacylglycerol to PG. It requires a divalent metal cation cofactor.
|
Metabolite
Metabolic
|
|
|
SMP0296283 |
Cardiolipin Biosynthesis CL(18:2(9Z,12Z)/20:4(5Z,8Z,11Z,14Z)/18:2(9Z,11Z)/18:1(9Z))Mus musculus
Cardiolipin (CL) is an important component of the inner mitochondrial membrane where it constitutes about 20% of the total lipid composition. It is essential for the optimal function of numerous enzymes that are involved in mitochondrial energy metabolism (Wikipedia). Cardiolipin biosynthesis occurs mainly in the mitochondria, but there also exists an alternative synthesis route for CDP-diacylglycerol that takes place in the endoplasmic reticulum. This second route may supplement this pathway. All membrane-localized enzymes are coloured dark green in the image. First, dihydroxyacetone phosphate (or glycerone phosphate) from glycolysis is used by the cytosolic enzyme glycerol-3-phosphate dehydrogenase [NAD(+)] to synthesize sn-glycerol 3-phosphate. Second, the mitochondrial outer membrane enzyme glycerol-3-phosphate acyltransferase esterifies an acyl-group to the sn-1 position of sn-glycerol 3-phosphate to form 1-acyl-sn-glycerol 3-phosphate (lysophosphatidic acid or LPA). Third, the enzyme 1-acyl-sn-glycerol-3-phosphate acyltransferase converts LPA into phosphatidic acid (PA or 1,2-diacyl-sn-glycerol 3-phosphate) by esterifying an acyl-group to the sn-2 position of the glycerol backbone. PA is then transferred to the inner mitochondrial membrane to continue cardiolipin synthesis. Fourth, magnesium-dependent phosphatidate cytidylyltransferase catalyzes the conversion of PA into CDP-diacylglycerol. Fifth, CDP-diacylglycerol--glycerol-3-phosphate 3-phosphatidyltransferase synthesizes phosphatidylglycerophosphate (PGP). Sixth, phosphatidylglycerophosphatase and protein-tyrosine phosphatase dephosphorylates PGP to form phosphatidylglycerol (PG). Last, cardiolipin synthase catalyzes the synthesis of cardiolipin by transferring a phosphatidyl group from a second CDP-diacylglycerol to PG. It requires a divalent metal cation cofactor.
|
Metabolite
Metabolic
|
|
|
SMP0402077![]() |
Glycolate and Glyoxylate DegradationBacteroides sp. 2_1_33B
Glycolic acid is introduced into the cytoplasm through either a glycolate / lactate:H+ symporter or a acetate / glycolate transporter. Once inside, glycolic acid reacts with an oxidized electron-transfer flavoprotein through a glycolate oxidase resulting in a reduced acceptor and glyoxylic acid. Glyoxylic acid can also be obtained from the introduction of glyoxylic acid. It can also be obtained from the metabolism of (S)-allantoin.
S-allantoin is introduced into the cytoplasm through a purine and pyrimidine transporter(allantoin specific). Once inside, the compound reacts with water through a allantoinase resulting in hydrogen ion and allantoic acid. Allantoic acid then reacts with water and hydrogen ion through a allantoate amidohydrolase resulting in a carbon dioxide, ammonium and S-ureidoglycine. The latter compound reacts with water through a S-ureidoglycine aminohydrolase resulting in ammonium and S-ureidoglycolic acid which in turn reacts with a Ureidoglycolate lyase resulting in urea and glyoxylic acid.
Glyoxylic acid can either be metabolized into L-malic acid by a reaction with acetyl-CoA and Water through a malate synthase G which also releases hydrogen ion and Coenzyme A. L-malic acid is then incorporated into the TCA cycle.
Glyoxylic acid can also be metabolized by glyoxylate carboligase, releasing a carbon dioxide and tartronate semialdehyde. The latter compound is then reduced by an NADH driven tartronate semialdehyde reductase 2 resulting in glyceric acid. Glyceric acid is phosphorylated by a glycerate kinase 2 resulting in a 3-phosphoglyceric acid. This compound is then integrated into various other pathways: cysteine biosynthesis, serine biosynthesis and glycolysis and pyruvate dehydrogenase.
|
Metabolite
Metabolic
|
|
Showing 349341 -
349350 of 351453 pathways