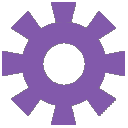
Browsing Pathways
Showing 349961 -
349970 of 605359 pathways
PathBank ID | Pathway Name and Description | Pathway Class | Chemical Compounds | Proteins |
---|---|---|---|---|
SMP0298077 |
Cardiolipin Biosynthesis CL(18:4(6Z,9Z,12Z,15Z)/18:2(9Z,12Z)/22:5(4Z,7Z,10Z,13Z,16Z)/18:1(9Z))Mus musculus
Cardiolipin (CL) is an important component of the inner mitochondrial membrane where it constitutes about 20% of the total lipid composition. It is essential for the optimal function of numerous enzymes that are involved in mitochondrial energy metabolism (Wikipedia). Cardiolipin biosynthesis occurs mainly in the mitochondria, but there also exists an alternative synthesis route for CDP-diacylglycerol that takes place in the endoplasmic reticulum. This second route may supplement this pathway. All membrane-localized enzymes are coloured dark green in the image. First, dihydroxyacetone phosphate (or glycerone phosphate) from glycolysis is used by the cytosolic enzyme glycerol-3-phosphate dehydrogenase [NAD(+)] to synthesize sn-glycerol 3-phosphate. Second, the mitochondrial outer membrane enzyme glycerol-3-phosphate acyltransferase esterifies an acyl-group to the sn-1 position of sn-glycerol 3-phosphate to form 1-acyl-sn-glycerol 3-phosphate (lysophosphatidic acid or LPA). Third, the enzyme 1-acyl-sn-glycerol-3-phosphate acyltransferase converts LPA into phosphatidic acid (PA or 1,2-diacyl-sn-glycerol 3-phosphate) by esterifying an acyl-group to the sn-2 position of the glycerol backbone. PA is then transferred to the inner mitochondrial membrane to continue cardiolipin synthesis. Fourth, magnesium-dependent phosphatidate cytidylyltransferase catalyzes the conversion of PA into CDP-diacylglycerol. Fifth, CDP-diacylglycerol--glycerol-3-phosphate 3-phosphatidyltransferase synthesizes phosphatidylglycerophosphate (PGP). Sixth, phosphatidylglycerophosphatase and protein-tyrosine phosphatase dephosphorylates PGP to form phosphatidylglycerol (PG). Last, cardiolipin synthase catalyzes the synthesis of cardiolipin by transferring a phosphatidyl group from a second CDP-diacylglycerol to PG. It requires a divalent metal cation cofactor.
|
Metabolite
Metabolic
|
|
|
SMP0399310![]() |
Chorismate BiosynthesisHelicobacter canadensis MIT 98-5491
Chorismate is an intermediate in tyrosine, phenylalanine and tryptophan synthesis and a precursor for folic acid, ubiquinone, enterochelin and menaquinone. Three enzymes catalyze the first step in chorismate biosynthesis. Synthesis may be reduced by feedback inhibition of tyrosine, phenylalanine and tryptophan to the enzymes. The biosynthesis of chorismate starts with D-Erythrose-4-phosphate getting transformed into 3-deoxy-D-arabino-heptulosonate-7-phosphate through a phospho-2-dehydro-3-deoxyheptonate aldolase. This is followed by a 3-dehydroquinate synthase converting the 3-deoxy-D-arabino-heptulosonate-7-phosphate into a 3-dehydroquinate which in turn is conveted to 3-dehydroshikimate through a 3-dehydroquinate dehydratase. At this point 3-dehydroshikimate can be turned into Shikimic acid through 2 different reactions involving Quinate/shikimate dehydrogenase and shikimate dehydrogenase 2. Shikimic acid is phosphorylated by Shikimate kinase 2 into shikimate 3-phosphate. Shikimate 3- phophate and a phosphoenolpyruvic acid are then joined through a 3-phosphoshikimate 1-carboxyvinyltransferase to produce a 5-enoylpyruvyl-shikimate 3-phosphate while releasing a phosphate. This in turn produces our final product Chorismate through a chorismate synthase.
|
Metabolite
Metabolic
|
|
|
SMP0394761![]() |
Histidine BiosynthesisHelicobacter canadensis MIT 98-5491
Histidine biosynthesis starts with a product of PRPP biosynthesis pathway, phosphoribosyl pyrophosphate which interacts with a hydrogen ion through an ATP phosphoribosyltransferase resulting in an pyrophosphate and a phosphoribosyl-ATP. The phosphoribosyl-ATP interacts with water through a phosphoribosyl-AMP cyclohydrolase / phosphoribosyl-ATP pyrophosphatase resulting in the release of pyrophosphate, hydrogen ion and a phosphoribosyl-AMP. The same enzyme proceeds to interact with phosphoribosyl-AMP and water resulting in a 1-(5'-Phosphoribosyl)-5-amino-4-imidazolecarboxamide. The product is then isomerized by a N-(5'-phospho-L-ribosyl-formimino)-5-amino-1-(5'-phosphoribosyl)-4-imidazolecarboxamide isomerase resulting in a PhosphoribosylformiminoAICAR-phosphate, which reacts with L-glutamine through an imidazole glycerol phosphate synthase resulting in a L-glutamic acid, hydrogen ion, 5-aminoimidazole-4-carboxamide and a D-erythro-imidazole-glycerol-phosphate. D-erythro-imidazole-glycerol-phosphate reacts with a imidazoleglycerol-phosphate dehydratase / histidinol-phosphatase, dehydrating the compound and resulting in a imidazole acetol-phosphate. Next, imidazole acetol-phosphate reacts with L-glutamic acid through a histidinol-phosphate aminotransferase, releasing oxoglutaric acid and L-histidinol-phosphate. The latter compound interacts with water and a imidazoleglycerol-phosphate dehydratase / histidinol-phosphatase resulting in L-histidinol and phosphate. L-histidinol interacts with a NAD-driven histidinol dehydrogenase resulting in a Histidinal. Histidinal in turn reacts with water in a NAD driven histidinal dehydrogenase resulting in L-Histidine.
L-Histidine then represses ATP phosphoribosyltransferase, regulation its own biosynthesis.
|
Metabolite
Metabolic
|
|
|
SMP0394770![]() |
Valine BiosynthesisCampylobacter upsaliensis JV21
The pathway of valine biosynthesis starts with pyruvic acid interacting with a hydrogen ion through a acetolactate synthase / acetohydroxybutanoate synthase or a acetohydroxybutanoate synthase / acetolactate synthase resulting in the release of carbon dioxide and (S)-2-acetolactate. The latter compound then interacts with a hydrogen ion through an NADPH driven
acetohydroxy acid isomeroreductase resulting in the release of a NADP and an (R) 2,3-dihydroxy-3-methylvalerate. The latter compound is then dehydrated by a dihydroxy acid dehydratase resulting in the release of water and isovaleric acid. Isovaleric acid interacts with an L-glutamic acid through a Valine Transaminase resulting in a oxoglutaric acid and an L-valine. L-valine is then transported into the periplasmic space through a L-valine efflux transporter.
|
Metabolite
Metabolic
|
|
|
SMP0394764![]() |
Leucine BiosynthesisHelicobacter bilis ATCC 43879
Leucine biosynthesis involves a five-step conversion process starting with the valine precursor 2-keto-isovalerate interacting with acetyl-CoA and water through a 2-isopropylmalate synthase resulting in Coenzyme A, hydrogen Ion and 2-isopropylmalic acid. The latter compound reacts with isopropylmalate isomerase which dehydrates the compound resulting in a Isopropylmaleate. This compound reacts with water through a isopropylmalate isomerase resulting in 3-isopropylmalate. This compound interacts with a NAD-driven D-malate / 3-isopropylmalate dehydrogenase results in 2-isopropyl-3-oxosuccinate. This compound interacts spontaneously with hydrogen resulting in the release of carbon dioxide and ketoleucine. Ketoleucine interacts in a reversible reaction with L-glutamic acid through a branched-chain amino-acid aminotransferase resulting in Oxoglutaric acid and L-leucine. L-leucine can then be exported outside the cytoplasm through a transporter: L-amino acid efflux transporter. In the final step, ketoleucine can be catalyzed to form L-leucine by branched-chain amino-acid aminotransferase (IlvE) and tyrosine aminotransferase (TryB). L-Glutamic acid can also be transformed into oxoglutaric acid by these two enzymes. Tyrosine aminotransferase can be suppressed by lecuine, and inhibited by 2-keto-isovarlerate and its end product, tyrosine. 2-ketoisocaproate can not be introduced if 2-keto-isovarlerate inhibit TyrB and IlvE is absent.
|
Metabolite
Metabolic
|
||
SMP0394768![]() |
Valine BiosynthesisHelicobacter canadensis MIT 98-5491
The pathway of valine biosynthesis starts with pyruvic acid interacting with a hydrogen ion through a acetolactate synthase / acetohydroxybutanoate synthase or a acetohydroxybutanoate synthase / acetolactate synthase resulting in the release of carbon dioxide and (S)-2-acetolactate. The latter compound then interacts with a hydrogen ion through an NADPH driven
acetohydroxy acid isomeroreductase resulting in the release of a NADP and an (R) 2,3-dihydroxy-3-methylvalerate. The latter compound is then dehydrated by a dihydroxy acid dehydratase resulting in the release of water and isovaleric acid. Isovaleric acid interacts with an L-glutamic acid through a Valine Transaminase resulting in a oxoglutaric acid and an L-valine. L-valine is then transported into the periplasmic space through a L-valine efflux transporter.
|
Metabolite
Metabolic
|
||
SMP0399432![]() |
Isoleucine BiosynthesisEnterobacter hormaechei YT2
Isoleucine biosynthesis begins with L-threonine from the threonine biosynthesis pathway. L-threonine interacts with threonine dehydratase biosynthetic releasing water, a hydrogen ion and (2Z)-2-aminobut-2-enoate. The latter is isomerized into a 2-iminobutanoate which interacts with water and a hydrogen ion spontaneously, resulting in the release of ammonium and 2-ketobutyric acid. 2-ketobutyric acid reacts with pyruvic acid and hydrogen ions through an acetohydroxybutanoate synthase / acetolactate synthase 2 resulting in carbon dioxide and (S)-2-Aceto-2-hydroxybutanoic acid. (S)-2-Aceto-2-hydroxybutanoic acid is reduced by an NADPH driven acetohydroxy acid isomeroreductase releasing NADP and acetohydroxy acid isomeroreductase. The latter compound is dehydrated by a dihydroxy acid dehydratase resulting in 3-methyl-2-oxovaleric acid. This compound reacts in a reversible reaction with L-glutamic acid through a Branched-chain-amino-acid aminotransferase resulting in oxoglutaric acid and L-isoleucine.
L-isoleucine can also be transported into the cytoplasm through two different methods: a branched chain amino acid ABC transporter or a branched chain amino acid transporter BrnQy.
|
Metabolite
Metabolic
|
|
|
SMP0394892![]() |
Histidine BiosynthesisEscherichia albertii TW07627
Histidine biosynthesis starts with a product of PRPP biosynthesis pathway, phosphoribosyl pyrophosphate which interacts with a hydrogen ion through an ATP phosphoribosyltransferase resulting in an pyrophosphate and a phosphoribosyl-ATP. The phosphoribosyl-ATP interacts with water through a phosphoribosyl-AMP cyclohydrolase / phosphoribosyl-ATP pyrophosphatase resulting in the release of pyrophosphate, hydrogen ion and a phosphoribosyl-AMP. The same enzyme proceeds to interact with phosphoribosyl-AMP and water resulting in a 1-(5'-Phosphoribosyl)-5-amino-4-imidazolecarboxamide. The product is then isomerized by a N-(5'-phospho-L-ribosyl-formimino)-5-amino-1-(5'-phosphoribosyl)-4-imidazolecarboxamide isomerase resulting in a PhosphoribosylformiminoAICAR-phosphate, which reacts with L-glutamine through an imidazole glycerol phosphate synthase resulting in a L-glutamic acid, hydrogen ion, 5-aminoimidazole-4-carboxamide and a D-erythro-imidazole-glycerol-phosphate. D-erythro-imidazole-glycerol-phosphate reacts with a imidazoleglycerol-phosphate dehydratase / histidinol-phosphatase, dehydrating the compound and resulting in a imidazole acetol-phosphate. Next, imidazole acetol-phosphate reacts with L-glutamic acid through a histidinol-phosphate aminotransferase, releasing oxoglutaric acid and L-histidinol-phosphate. The latter compound interacts with water and a imidazoleglycerol-phosphate dehydratase / histidinol-phosphatase resulting in L-histidinol and phosphate. L-histidinol interacts with a NAD-driven histidinol dehydrogenase resulting in a Histidinal. Histidinal in turn reacts with water in a NAD driven histidinal dehydrogenase resulting in L-Histidine.
L-Histidine then represses ATP phosphoribosyltransferase, regulation its own biosynthesis.
|
Metabolite
Metabolic
|
|
|
SMP0405827![]() |
Galactose MetabolismPrevotella oralis ATCC 33269
Galactose can be synthesized through two pathways: melibiose degradation involving an alpha galactosidase and lactose degradation involving a beta galactosidase. Melibiose is first transported inside the cell through the melibiose:Li+/Na+/H+ symporter. Once inside the cell, melibiose is degraded through alpha galactosidase into an alpha-D-galactose and a beta-D-glucose. The beta-D-glucose is phosphorylated by a glucokinase to produce a beta-D-glucose-6-phosphate which can spontaneously be turned into a alpha D glucose 6 phosphate. This alpha D-glucose-6-phosphate is metabolized into a glucose -1-phosphate through a phosphoglucomutase-1. The glucose -1-phosphate is transformed into a uridine diphosphate glucose through UTP--glucose-1-phosphate uridylyltransferase. The product, uridine diphosphate glucose, can undergo a reversible reaction in which it can be turned into uridine diphosphategalactose through an UDP-glucose 4-epimerase.
Galactose can also be produced by lactose degradation involving a lactose permease to uptake lactose from the environment and a beta-galactosidase to turn lactose into Beta-D-galactose.
Beta-D-galactose can also be uptaken from the environment through a galactose proton symporter.
Galactose is degraded through the following process:
Beta-D-galactose is introduced into the cytoplasm through a galactose proton symporter, or it can be synthesized from an alpha lactose that is introduced into the cytoplasm through a lactose permease. Alpha lactose interacts with water through a beta-galactosidase resulting in a beta-D-glucose and beta-D-galactose. Beta-D-galactose is isomerized into D-galactose. D-Galactose undergoes phosphorylation through a galactokinase, hence producing galactose 1 phosphate. On the other side of the pathway, a gluose-1-phosphate (product of the interaction of alpha-D-glucose 6-phosphate with a phosphoglucomutase resulting in a alpha-D-glucose-1-phosphate, an isomer of Glucose 1-phosphate, or an isomer of Beta-D-glucose 1-phosphate) interacts with UTP and a hydrogen ion in order to produce a uridine diphosphate glucose. This is followed by the interaction of galactose-1-phosphate with an established amount of uridine diphosphate glucose through a galactose-1-phosphate uridylyltransferase, which in turn output a glucose-1-phosphate and a uridine diphosphate galactose. The glucose -1-phosphate is transformed into a uridine diphosphate glucose through UTP--glucose-1-phosphate uridylyltransferase. The product, uridine diphosphate glucose, can undergo a reversible reaction in which it can be turned into uridine diphosphategalactose through an UDP-glucose 4-epimerase, and so the cycle can keep going as long as more lactose or galactose is imported into the cell
|
Metabolite
Metabolic
|
|
|
SMP0298101 |
Cardiolipin Biosynthesis CL(18:4(6Z,9Z,12Z,15Z)/18:2(9Z,12Z)/22:6(4Z,7Z,10Z,13Z,16Z,19Z)/14:0)Mus musculus
Cardiolipin (CL) is an important component of the inner mitochondrial membrane where it constitutes about 20% of the total lipid composition. It is essential for the optimal function of numerous enzymes that are involved in mitochondrial energy metabolism (Wikipedia). Cardiolipin biosynthesis occurs mainly in the mitochondria, but there also exists an alternative synthesis route for CDP-diacylglycerol that takes place in the endoplasmic reticulum. This second route may supplement this pathway. All membrane-localized enzymes are coloured dark green in the image. First, dihydroxyacetone phosphate (or glycerone phosphate) from glycolysis is used by the cytosolic enzyme glycerol-3-phosphate dehydrogenase [NAD(+)] to synthesize sn-glycerol 3-phosphate. Second, the mitochondrial outer membrane enzyme glycerol-3-phosphate acyltransferase esterifies an acyl-group to the sn-1 position of sn-glycerol 3-phosphate to form 1-acyl-sn-glycerol 3-phosphate (lysophosphatidic acid or LPA). Third, the enzyme 1-acyl-sn-glycerol-3-phosphate acyltransferase converts LPA into phosphatidic acid (PA or 1,2-diacyl-sn-glycerol 3-phosphate) by esterifying an acyl-group to the sn-2 position of the glycerol backbone. PA is then transferred to the inner mitochondrial membrane to continue cardiolipin synthesis. Fourth, magnesium-dependent phosphatidate cytidylyltransferase catalyzes the conversion of PA into CDP-diacylglycerol. Fifth, CDP-diacylglycerol--glycerol-3-phosphate 3-phosphatidyltransferase synthesizes phosphatidylglycerophosphate (PGP). Sixth, phosphatidylglycerophosphatase and protein-tyrosine phosphatase dephosphorylates PGP to form phosphatidylglycerol (PG). Last, cardiolipin synthase catalyzes the synthesis of cardiolipin by transferring a phosphatidyl group from a second CDP-diacylglycerol to PG. It requires a divalent metal cation cofactor.
|
Metabolite
Metabolic
|
|
Showing 349961 -
349970 of 350057 pathways