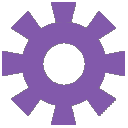
Browsing Pathways
Showing 350351 -
350360 of 605359 pathways
PathBank ID | Pathway Name and Description | Pathway Class | Chemical Compounds | Proteins |
---|---|---|---|---|
SMP0395280![]() |
Leucine BiosynthesisPhascolarctobacterium succinatutens YIT 12067
Leucine biosynthesis involves a five-step conversion process starting with the valine precursor 2-keto-isovalerate interacting with acetyl-CoA and water through a 2-isopropylmalate synthase resulting in Coenzyme A, hydrogen Ion and 2-isopropylmalic acid. The latter compound reacts with isopropylmalate isomerase which dehydrates the compound resulting in a Isopropylmaleate. This compound reacts with water through a isopropylmalate isomerase resulting in 3-isopropylmalate. This compound interacts with a NAD-driven D-malate / 3-isopropylmalate dehydrogenase results in 2-isopropyl-3-oxosuccinate. This compound interacts spontaneously with hydrogen resulting in the release of carbon dioxide and ketoleucine. Ketoleucine interacts in a reversible reaction with L-glutamic acid through a branched-chain amino-acid aminotransferase resulting in Oxoglutaric acid and L-leucine. L-leucine can then be exported outside the cytoplasm through a transporter: L-amino acid efflux transporter. In the final step, ketoleucine can be catalyzed to form L-leucine by branched-chain amino-acid aminotransferase (IlvE) and tyrosine aminotransferase (TryB). L-Glutamic acid can also be transformed into oxoglutaric acid by these two enzymes. Tyrosine aminotransferase can be suppressed by lecuine, and inhibited by 2-keto-isovarlerate and its end product, tyrosine. 2-ketoisocaproate can not be introduced if 2-keto-isovarlerate inhibit TyrB and IlvE is absent.
|
Metabolite
Metabolic
|
||
SMP0395302![]() |
Valine BiosynthesisSelenomonas ruminantium AC2024
The pathway of valine biosynthesis starts with pyruvic acid interacting with a hydrogen ion through a acetolactate synthase / acetohydroxybutanoate synthase or a acetohydroxybutanoate synthase / acetolactate synthase resulting in the release of carbon dioxide and (S)-2-acetolactate. The latter compound then interacts with a hydrogen ion through an NADPH driven
acetohydroxy acid isomeroreductase resulting in the release of a NADP and an (R) 2,3-dihydroxy-3-methylvalerate. The latter compound is then dehydrated by a dihydroxy acid dehydratase resulting in the release of water and isovaleric acid. Isovaleric acid interacts with an L-glutamic acid through a Valine Transaminase resulting in a oxoglutaric acid and an L-valine. L-valine is then transported into the periplasmic space through a L-valine efflux transporter.
|
Metabolite
Metabolic
|
||
SMP0400084![]() |
Chorismate BiosynthesisMitsuokella multacida DSM 20544
Chorismate is an intermediate in tyrosine, phenylalanine and tryptophan synthesis and a precursor for folic acid, ubiquinone, enterochelin and menaquinone. Three enzymes catalyze the first step in chorismate biosynthesis. Synthesis may be reduced by feedback inhibition of tyrosine, phenylalanine and tryptophan to the enzymes. The biosynthesis of chorismate starts with D-Erythrose-4-phosphate getting transformed into 3-deoxy-D-arabino-heptulosonate-7-phosphate through a phospho-2-dehydro-3-deoxyheptonate aldolase. This is followed by a 3-dehydroquinate synthase converting the 3-deoxy-D-arabino-heptulosonate-7-phosphate into a 3-dehydroquinate which in turn is conveted to 3-dehydroshikimate through a 3-dehydroquinate dehydratase. At this point 3-dehydroshikimate can be turned into Shikimic acid through 2 different reactions involving Quinate/shikimate dehydrogenase and shikimate dehydrogenase 2. Shikimic acid is phosphorylated by Shikimate kinase 2 into shikimate 3-phosphate. Shikimate 3- phophate and a phosphoenolpyruvic acid are then joined through a 3-phosphoshikimate 1-carboxyvinyltransferase to produce a 5-enoylpyruvyl-shikimate 3-phosphate while releasing a phosphate. This in turn produces our final product Chorismate through a chorismate synthase.
|
Metabolite
Metabolic
|
|
|
SMP0395285![]() |
Valine Biosynthesis[Bacteroides] pectinophilus ATCC 43243
The pathway of valine biosynthesis starts with pyruvic acid interacting with a hydrogen ion through a acetolactate synthase / acetohydroxybutanoate synthase or a acetohydroxybutanoate synthase / acetolactate synthase resulting in the release of carbon dioxide and (S)-2-acetolactate. The latter compound then interacts with a hydrogen ion through an NADPH driven
acetohydroxy acid isomeroreductase resulting in the release of a NADP and an (R) 2,3-dihydroxy-3-methylvalerate. The latter compound is then dehydrated by a dihydroxy acid dehydratase resulting in the release of water and isovaleric acid. Isovaleric acid interacts with an L-glutamic acid through a Valine Transaminase resulting in a oxoglutaric acid and an L-valine. L-valine is then transported into the periplasmic space through a L-valine efflux transporter.
|
Metabolite
Metabolic
|
||
SMP0405954![]() |
NAD SalvageParabacteroides sp. 20_3
NAD molecules have a relatively short half-life. NAD can be degraded by enzymes, and the degraded NAD molecule can be recouped by NAD salvage cycles. NAD salvage cycles can be used for recycling degraded NAD products such as nicotinamide and nicotinamide D-ribonucleotide. NAD salvage cycles can also be used for absorption of exogenous NAD+. NAD reacts spontaneously with water resulting in the release of hydrogen ion, AMP and beta-nicotinamide D-ribonucleotide. This enzyme can either interact spontaneously with water resulting in the release of D-ribofuranose 5-phosphate, hydrogen ion and Nacinamide. On the other hand beta-nicotinamide D-ribonucleotide can also react with water through NMN amidohydrolase resulting in ammonium, and Nicotinate beta-D-ribonucleotide. Also it can interact with water spontaneously resulting in the release of phosphate resulting in a Nicotinamide riboside. Niacinamide interacts with water through a nicotinamidase resulting in a release of ammonium and nicotinic acid. Nicotinic acid interacts with water and phosphoribosyl pyrophosphate through an ATP driven nicotinate phosphoribosyltransferase resulting in the release of ADP, pyrophosphate and phosphate and nicotinate beta-D-ribonucleotide. Nicotinamide riboside interacts with an ATP driven NadR DNA-binding transcriptional repressor and NMN adenylyltransferase (Escherichia coli) resulting in a ADP, hydrogen ion and beta-nicotinamide D-ribonucleotide. The latter interacts with ATP and hydrogen ions through NadR DNA-binding transcriptional repressor and NMN adenylyltransferase resulting in pyrophosphate and NAD. Nicotinate beta-D-ribonucleotide is adenylated through the interaction with ATP and a hydrogen ion through a nicotinate-mononucleotide adenylyltransferase resulting in pyrophosphate and Nicotinic acid adenine dinucleotide. Nicotinic acid adenine dinucleotide interacts with L-glutamine and water through an ATP driven NAD synthease, NH3-dependent resulting in AMP, pyrophosphate, hydrogen ion, L-glutamic acid and NAD.
|
Metabolite
Metabolic
|
|
|
SMP0405952![]() |
NAD BiosynthesisParabacteroides sp. 20_3
Nicotinamide adenine dinucleotide (NAD) can be biosynthesized from L-aspartic acid. This amino acid reacts with oxygen through an L-aspartate oxidase resulting in a hydrogen ion, hydrogen peroxide and an iminoaspartic acid. The latter compound interacts with dihydroxyacetone phosphate through a quinolinate synthase A, resulting in a phosphate, water, and a quinolic acid. Quinolic acid interacts with phosphoribosyl pyrophosphate and hydrogen ion through a quinolinate phosphoribosyltransferase resulting in pyrophosphate, carbon dioxide and nicotinate beta-D-ribonucleotide. The latter is adenylated through an ATP driven nicotinate-mononucleotide adenylyltransferase releasing a pyrophosphate and resulting in a nicotinic acid adenine dinucleotide.
Nicotinic acid adenine dinucleotide is processed through an NAD synthetase, NH3-dependent in two different manners.
In the first case, Nicotinic acid adenine dinucleotide interacts with ATP, L-glutamine and water through the enzyme and results in hydrogen ion, AMP, pyrophosphate, L-glutamic acid and NAD.
In the second case, Nicotinic acid adenine dinucleotide interacts with ATP and ammonium through the enzyme resulting in a pyrophosphate, AMP, hydrogen ion and NAD.
NAD then proceeds to regulate its own pathway by repressing L-aspartate oxidase.
As a general rule, most prokaryotes utilize the aspartate de novo pathway, in which the nicotinate moiety of NAD is synthesized from aspartate , while in eukaryotes, the de novo pathway starts with tryptophan.
|
Metabolite
Metabolic
|
|
|
SMP0298260 |
Cardiolipin Biosynthesis CL(18:4(6Z,9Z,12Z,15Z)/18:4(6Z,9Z,12Z,15Z)/20:5(5Z,8Z,11Z,14Z,17Z)/18:2(9Z,12Z))Mus musculus
Cardiolipin (CL) is an important component of the inner mitochondrial membrane where it constitutes about 20% of the total lipid composition. It is essential for the optimal function of numerous enzymes that are involved in mitochondrial energy metabolism (Wikipedia). Cardiolipin biosynthesis occurs mainly in the mitochondria, but there also exists an alternative synthesis route for CDP-diacylglycerol that takes place in the endoplasmic reticulum. This second route may supplement this pathway. All membrane-localized enzymes are coloured dark green in the image. First, dihydroxyacetone phosphate (or glycerone phosphate) from glycolysis is used by the cytosolic enzyme glycerol-3-phosphate dehydrogenase [NAD(+)] to synthesize sn-glycerol 3-phosphate. Second, the mitochondrial outer membrane enzyme glycerol-3-phosphate acyltransferase esterifies an acyl-group to the sn-1 position of sn-glycerol 3-phosphate to form 1-acyl-sn-glycerol 3-phosphate (lysophosphatidic acid or LPA). Third, the enzyme 1-acyl-sn-glycerol-3-phosphate acyltransferase converts LPA into phosphatidic acid (PA or 1,2-diacyl-sn-glycerol 3-phosphate) by esterifying an acyl-group to the sn-2 position of the glycerol backbone. PA is then transferred to the inner mitochondrial membrane to continue cardiolipin synthesis. Fourth, magnesium-dependent phosphatidate cytidylyltransferase catalyzes the conversion of PA into CDP-diacylglycerol. Fifth, CDP-diacylglycerol--glycerol-3-phosphate 3-phosphatidyltransferase synthesizes phosphatidylglycerophosphate (PGP). Sixth, phosphatidylglycerophosphatase and protein-tyrosine phosphatase dephosphorylates PGP to form phosphatidylglycerol (PG). Last, cardiolipin synthase catalyzes the synthesis of cardiolipin by transferring a phosphatidyl group from a second CDP-diacylglycerol to PG. It requires a divalent metal cation cofactor.
|
Metabolite
Metabolic
|
|
|
SMP0405959![]() |
Glutathione MetabolismParabacteroides sp. 20_3
The biosynthesis of glutathione starts with the introduction of L-glutamic acid through either a glutamate:sodium symporter, glutamate / aspartate : H+ symporter GltP or a
glutamate / aspartate ABC transporter. Once in the cytoplasm, L-glutamice acid reacts with L-cysteine through an ATP glutamate-cysteine ligase resulting in gamma-glutamylcysteine. This compound reacts which Glycine through an ATP driven glutathione synthetase thus catabolizing Glutathione.
This compound is metabolized through a spontaneous reaction with an oxidized glutaredoxin resulting in a reduced glutaredoxin and an oxidized glutathione. This compound is reduced by a NADPH glutathione reductase resulting in a glutathione.
Glutathione can then be degraded into various different glutathione containing compounds by reacting with a napthalene or Bromobenzene-2,3-oxide through a glutathione S-transferase.
|
Metabolite
Metabolic
|
|
|
SMP0298252 |
Cardiolipin Biosynthesis CL(18:4(6Z,9Z,12Z,15Z)/18:4(6Z,9Z,12Z,15Z)/20:4(5Z,8Z,11Z,14Z)/22:5(4Z,7Z,10Z,13Z,16Z))Mus musculus
Cardiolipin (CL) is an important component of the inner mitochondrial membrane where it constitutes about 20% of the total lipid composition. It is essential for the optimal function of numerous enzymes that are involved in mitochondrial energy metabolism (Wikipedia). Cardiolipin biosynthesis occurs mainly in the mitochondria, but there also exists an alternative synthesis route for CDP-diacylglycerol that takes place in the endoplasmic reticulum. This second route may supplement this pathway. All membrane-localized enzymes are coloured dark green in the image. First, dihydroxyacetone phosphate (or glycerone phosphate) from glycolysis is used by the cytosolic enzyme glycerol-3-phosphate dehydrogenase [NAD(+)] to synthesize sn-glycerol 3-phosphate. Second, the mitochondrial outer membrane enzyme glycerol-3-phosphate acyltransferase esterifies an acyl-group to the sn-1 position of sn-glycerol 3-phosphate to form 1-acyl-sn-glycerol 3-phosphate (lysophosphatidic acid or LPA). Third, the enzyme 1-acyl-sn-glycerol-3-phosphate acyltransferase converts LPA into phosphatidic acid (PA or 1,2-diacyl-sn-glycerol 3-phosphate) by esterifying an acyl-group to the sn-2 position of the glycerol backbone. PA is then transferred to the inner mitochondrial membrane to continue cardiolipin synthesis. Fourth, magnesium-dependent phosphatidate cytidylyltransferase catalyzes the conversion of PA into CDP-diacylglycerol. Fifth, CDP-diacylglycerol--glycerol-3-phosphate 3-phosphatidyltransferase synthesizes phosphatidylglycerophosphate (PGP). Sixth, phosphatidylglycerophosphatase and protein-tyrosine phosphatase dephosphorylates PGP to form phosphatidylglycerol (PG). Last, cardiolipin synthase catalyzes the synthesis of cardiolipin by transferring a phosphatidyl group from a second CDP-diacylglycerol to PG. It requires a divalent metal cation cofactor.
|
Metabolite
Metabolic
|
|
|
SMP0298265 |
Cardiolipin Biosynthesis CL(18:4(6Z,9Z,12Z,15Z)/18:4(6Z,9Z,12Z,15Z)/20:5(5Z,8Z,11Z,14Z,17Z)/20:5(5Z,8Z,11Z,14Z,17Z))Mus musculus
Cardiolipin (CL) is an important component of the inner mitochondrial membrane where it constitutes about 20% of the total lipid composition. It is essential for the optimal function of numerous enzymes that are involved in mitochondrial energy metabolism (Wikipedia). Cardiolipin biosynthesis occurs mainly in the mitochondria, but there also exists an alternative synthesis route for CDP-diacylglycerol that takes place in the endoplasmic reticulum. This second route may supplement this pathway. All membrane-localized enzymes are coloured dark green in the image. First, dihydroxyacetone phosphate (or glycerone phosphate) from glycolysis is used by the cytosolic enzyme glycerol-3-phosphate dehydrogenase [NAD(+)] to synthesize sn-glycerol 3-phosphate. Second, the mitochondrial outer membrane enzyme glycerol-3-phosphate acyltransferase esterifies an acyl-group to the sn-1 position of sn-glycerol 3-phosphate to form 1-acyl-sn-glycerol 3-phosphate (lysophosphatidic acid or LPA). Third, the enzyme 1-acyl-sn-glycerol-3-phosphate acyltransferase converts LPA into phosphatidic acid (PA or 1,2-diacyl-sn-glycerol 3-phosphate) by esterifying an acyl-group to the sn-2 position of the glycerol backbone. PA is then transferred to the inner mitochondrial membrane to continue cardiolipin synthesis. Fourth, magnesium-dependent phosphatidate cytidylyltransferase catalyzes the conversion of PA into CDP-diacylglycerol. Fifth, CDP-diacylglycerol--glycerol-3-phosphate 3-phosphatidyltransferase synthesizes phosphatidylglycerophosphate (PGP). Sixth, phosphatidylglycerophosphatase and protein-tyrosine phosphatase dephosphorylates PGP to form phosphatidylglycerol (PG). Last, cardiolipin synthase catalyzes the synthesis of cardiolipin by transferring a phosphatidyl group from a second CDP-diacylglycerol to PG. It requires a divalent metal cation cofactor.
|
Metabolite
Metabolic
|
|
Showing 350351 -
350360 of 351207 pathways