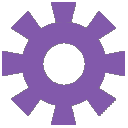
Browsing Pathways
Showing 355041 -
355050 of 605359 pathways
PathBank ID | Pathway Name and Description | Pathway Class | Chemical Compounds | Proteins |
---|---|---|---|---|
SMP0412501![]() |
Lipopolysaccharide BiosynthesisEscherichia coli 536
E. coli lipid A is synthesized on the cytoplasmic surface of the inner membrane. Starting with either the fructose 6-phosphate produced by glycolysis and pyruvate dehydrogenase or obtained from the interaction with D-fructose interacting with a mannose PTS permease. Fructose 6-phosphate interacts with L-glutamine through a D-fructose-6-phosphate aminotransferase resulting into a L-glutamic acid and a glucosamine 6-phosphate. The latter compound is isomerized through a phosphoglucosamine mutase resulting a glucosamine 1-phosphate. This compound is acetylated, interacting with acetyl-CoA through a bifunctional protein glmU resulting in a Coenzyme A, hydrogen ion and N-acetyl-glucosamine 1-phosphate. The latter interacts with UTP and hydrogen ion through the bifunctional protein glmU resulting in a pyrophosphate and a UDP-N-acetylglucosamine. UDP-N-acetylglucosamine iinteracts with (3R)-3-hydroxymyristoyl-[acp] through an UDP-N-acetylglucosamine acyltransferase resulting in a holo-[acp] and a UDP-3-O[(3R)-3-hydroxymyristoyl]-N-acetyl-alpha-D-glucosamine. The latter continues and reacts with water through UDP-3-O-acyl-N-acetylglucosamine deacetylase resulting in an acetic acid and UDP-3-O-(3-hydroxymyristoyl)-α-D-glucosamine. The latter compound interacts with (3R)-3-hydroxymyristoyl-[acp] through
UDP-3-O-(R-3-hydroxymyristoyl)-glucosamine N-acyltransferase releasing a hydrogen ion, a holo-acp and UDP-2-N,3-O-bis[(3R)-3-hydroxytetradecanoyl]-α-D-glucosamine. The latter compound is hydrolase by interacting with water and a UDP-2,3-diacylglucosamine hydrolase resulting in UMP, hydrogen ion and 2,3-bis[(3R)-3-hydroxymyristoyl]-α-D-glucosaminyl 1-phosphate. The latter interacts with a UDP-2-N,3-O-bis[(3R)-3-hydroxytetradecanoyl]-α-D-glucosamine through a lipid A disaccharide synthase resulting in a release of UDP, hydrogen ion and a lipid A disaccharide. The lipid A disaccharide is phosphorylated by an ATP mediated tetraacyldisaccharide 4'-kinase resulting in the release of hydrogen ion and lipid IVA. A D-ribulose 5-phosphate is isomerized with D-arabinose 5-phosphate isomerase 2 resulting in a D-arabinose 5-phosphate. D-arabinose 5-phosphate interacts with water and phosphoenolpyruvic acid through a 3-deoxy-D-manno-octulosonate 8-phosphate synthase resulting in the release of phosphate and 3-deoxy-D-manno-octulosonate 8-phosphate. This compound interacts with water through a 3-deoxy-D-manno-octulosonate 8-phosphate phosphatase thus releasing a phosphate and a 3-deoxy-D-manno-octulosonate. The latter compound interacts with CTP through a 3-deoxy-D-manno-octulosonate cytidylyltransferase resulting in a pyrophosphate and CMP-3-deoxy-α-D-manno-octulosonate.
CMP-3-deoxy-α-D-manno-octulosonate and lipid IVA interact with each other through a KDO transferase resulting in CMP, hydrogen ion and alpha-Kdo-(2-->6)-lipid IVA. The latter compound reacts with CMP-3-deoxy-α-D-manno-octulosonate through a KDO transferase resulting in a CMP, hydrogen ion, and a a-Kdo-(2->4)-a-Kdo-(2->6)-lipid IVA. The latter compound can either react with a palmitoleoyl-acp through a palmitoleoyl acyltransferase resulting in the release of a holo-acyl carriere protein and a Kdo2-palmitoleoyl-lipid IVa which in turn reacts with a myristoyl-acp through a myristoyl-acp dependent acyltransferase resulting in a release of a holo-acp and a Kdo2-lipid A, cold adapted, or it can interact with a dodecanoyl-[acp] lauroyl acyltransferase resulting in a holo-[acp] and a (KDO)2-(lauroyl)-lipid IVA. The latter compound reacts with a myristoyl-[acp] through a myristoyl-acyl carrier protein (ACP)-dependent acyltransferase resulting in a holo-[acp], (KDO)2-lipid A. The latter compound reacts with ADP-L-glycero-beta-D-manno-heptose through ADP-heptose:LPS heptosyltransferase I resulting hydrogen ion, ADP, heptosyl-KDO2-lipid A. The latter compound interacts with ADP-L-glycero-beta-D-manno-heptose through ADP-heptose:LPS heptosyltransferase II resulting in ADP, hydrogen ion and (heptosyl)2-Kdo2-lipid A. The latter compound UDP-glucose interacts with (heptosyl)2-Kdo2-lipid A resulting in UDP, hydrogen ion and glucosyl-(heptosyl)2-Kdo2-lipid A. Glucosyl-(heptosyl)2-Kdo2-lipid A (Escherichia coli) is phosphorylated through an ATP-mediated lipopolysaccharide core heptose (I) kinase resulting in ADP, hydrogen ion and glucosyl-(heptosyl)2-Kdo2-lipid A-phosphate.
The latter compound interacts with ADP-L-glycero-beta-D-manno-heptose through a lipopolysaccharide core heptosyl transferase III resulting in ADP, hydrogen ion, and glucosyl-(heptosyl)3-Kdo2-lipid A-phosphate. The latter compound is phosphorylated through an ATP-driven lipopolysaccharide core heptose (II) kinase resulting in ADP, hydrogen ion and glucosyl-(heptosyl)3-Kdo2-lipid A-bisphosphate. The latter compound interacts with UDP-alpha-D-galactose through a UDP-D-galactose:(glucosyl)lipopolysaccharide-1,6-D-galactosyltransferase resulting in a UDP, a hydrogen ion and a galactosyl-glucosyl-(heptosyl)3-Kdo2-lipid A-bisphosphate. The latter compound interacts with UDP-glucose through a (glucosyl)LPS α-1,3-glucosyltransferase resulting in a hydrogen ion, a UDP and galactosyl-(glucosyl)2-(heptosyl)3-Kdo2-lipid A-bisphosphate. This compound then interacts with UDP-glucose through a UDP-glucose:(glucosyl)LPS α-1,2-glucosyltransferase resulting in UDP, a hydrogen ion and galactosyl-(glucosyl)3-(heptosyl)3-Kdo2-lipid A-bisphosphate. This compound then interacts with ADP-L-glycero-beta-D-manno-heptose through a lipopolysaccharide core biosynthesis; heptosyl transferase IV; probably hexose transferase resulting in a Lipid A-core.
A lipid A-core is then exported into the periplasmic space by a lipopolysaccharide ABC transporter.
The lipid A-core is then flipped to the outer surface of the inner membrane by the ATP-binding cassette (ABC) transporter, MsbA. An additional integral membrane protein, YhjD, has recently been implicated in LPS export across the IM. The smallest LPS derivative that supports viability in E. coli is lipid IVA. However, it requires mutations in either MsbA or YhjD, to suppress the normally lethal consequence of an incomplete lipid A . Recent studies with deletion mutants implicate the periplasmic protein LptA, the cytosolic protein LptB, and the IM proteins LptC, LptF, and LptG in the subsequent transport of nascent LPS to the outer membrane (OM), where the LptD/LptE complex flips LPS to the outer surface.
|
Metabolite
Metabolic
|
|
|
SMP0412494![]() |
Fucose and Rhamnose DegradationEscherichia coli 536
In E. coli, L-fucose and L-rhamnose are metabolized through parallel pathways. The pathways converge after their corresponding aldolase reactions yielding the same products: lactaldehye. Proton symporter can facilitate the import of alpha-L-rhamnopyranose, methylpentose and beta-L-rhamnopyranose into cell for further metabolism, which allow E.coli to grow with carbon and energy. For alpha-L-rhamnopyranose, it is isomerized by a l-rhamnose mutarotase resulting in a beta-L-rhamnopyranose which is then isomerized into a keto-L-rhamnulose by a l-rhamnose isomerase. The keto-L-rhamnulose spontaneously changes into a L-rhamnulofuranose which is phosphorylated by a rhamnulokinase resulting in a L-rhamnulose 1-phosphate. This compound reacts with a rhamnulose-1-phosphate aldolase resulting in a dihydroxyacetone phosphate and a lactaldehyde. For beta-L-rhamnopyranose, it is isomerized by a L-fucose mutarotase resulting in a alpha-L-fucopyranose. This compound is then isomerized by an L-fucose isomerase resulting in a L-fuculose which in turn gets phosphorylated into an L-fuculose 1-phosphate through an L-fuculokinase. The compound L-fuculose 1-phosphate reacts with an L-fuculose phosphate aldolase through a dihydroxyacetone phosphate and a lactaldehyde. Two pathways can both be used for degrading L-lactaldehyde, which the aerobic pathway facilitates the conversion from L-lactic acid to pyruvic acid via L-lactate dehydrogenase, and the anaerobic pathway facilitates conversion from lactaldehyde to propane-1,2-diol via lactaldehyde reductase. Under aerobic conditions, L-lactaldehyde is oxidized in two steps to pyruvate, thereby channeling all the carbons from fucose or rhamnose into central metabolic pathways. Under anaerobic conditions, L-lactaldehyde is reduced to L-1,2-propanediol, which is secreted into the environment.
|
Metabolite
Metabolic
|
|
|
SMP0354509![]() |
D-Allulose DegradationEscherichia coli 536
D-allose can be used as source of carbon for E.coli. D-allose is imported into E.coli by D-allose ABC transporter without phosphorylation. Allose-6-phosphate isomerase and allulose-6-phosphate 3-epimerase catalyze the remaining reactions resulting in D-allulose 6 phosphate and Beta-D-fructofuranose 6-phosphate respectively. Once Beta D fructofuranose 6-phosphate is synthesized, it can be used in the glycolysis and pyruvatedehydrogenase pathway.
|
Metabolite
Metabolic
|
||
SMP0303604 |
Cardiolipin Biosynthesis CL(13:0/14:0/18:2(9Z,11Z)/19:0)Mus musculus
Cardiolipin (CL) is an important component of the inner mitochondrial membrane where it constitutes about 20% of the total lipid composition. It is essential for the optimal function of numerous enzymes that are involved in mitochondrial energy metabolism (Wikipedia). Cardiolipin biosynthesis occurs mainly in the mitochondria, but there also exists an alternative synthesis route for CDP-diacylglycerol that takes place in the endoplasmic reticulum. This second route may supplement this pathway. All membrane-localized enzymes are coloured dark green in the image. First, dihydroxyacetone phosphate (or glycerone phosphate) from glycolysis is used by the cytosolic enzyme glycerol-3-phosphate dehydrogenase [NAD(+)] to synthesize sn-glycerol 3-phosphate. Second, the mitochondrial outer membrane enzyme glycerol-3-phosphate acyltransferase esterifies an acyl-group to the sn-1 position of sn-glycerol 3-phosphate to form 1-acyl-sn-glycerol 3-phosphate (lysophosphatidic acid or LPA). Third, the enzyme 1-acyl-sn-glycerol-3-phosphate acyltransferase converts LPA into phosphatidic acid (PA or 1,2-diacyl-sn-glycerol 3-phosphate) by esterifying an acyl-group to the sn-2 position of the glycerol backbone. PA is then transferred to the inner mitochondrial membrane to continue cardiolipin synthesis. Fourth, magnesium-dependent phosphatidate cytidylyltransferase catalyzes the conversion of PA into CDP-diacylglycerol. Fifth, CDP-diacylglycerol--glycerol-3-phosphate 3-phosphatidyltransferase synthesizes phosphatidylglycerophosphate (PGP). Sixth, phosphatidylglycerophosphatase and protein-tyrosine phosphatase dephosphorylates PGP to form phosphatidylglycerol (PG). Last, cardiolipin synthase catalyzes the synthesis of cardiolipin by transferring a phosphatidyl group from a second CDP-diacylglycerol to PG. It requires a divalent metal cation cofactor.
|
Metabolite
Metabolic
|
||
SMP0412489![]() |
Galactose MetabolismEscherichia coli 536
Galactose can be synthesized through two pathways: melibiose degradation involving an alpha galactosidase and lactose degradation involving a beta galactosidase. Melibiose is first transported inside the cell through the melibiose:Li+/Na+/H+ symporter. Once inside the cell, melibiose is degraded through alpha galactosidase into an alpha-D-galactose and a beta-D-glucose. The beta-D-glucose is phosphorylated by a glucokinase to produce a beta-D-glucose-6-phosphate which can spontaneously be turned into a alpha D glucose 6 phosphate. This alpha D-glucose-6-phosphate is metabolized into a glucose -1-phosphate through a phosphoglucomutase-1. The glucose -1-phosphate is transformed into a uridine diphosphate glucose through UTP--glucose-1-phosphate uridylyltransferase. The product, uridine diphosphate glucose, can undergo a reversible reaction in which it can be turned into uridine diphosphategalactose through an UDP-glucose 4-epimerase.
Galactose can also be produced by lactose degradation involving a lactose permease to uptake lactose from the environment and a beta-galactosidase to turn lactose into Beta-D-galactose.
Beta-D-galactose can also be uptaken from the environment through a galactose proton symporter.
Galactose is degraded through the following process:
Beta-D-galactose is introduced into the cytoplasm through a galactose proton symporter, or it can be synthesized from an alpha lactose that is introduced into the cytoplasm through a lactose permease. Alpha lactose interacts with water through a beta-galactosidase resulting in a beta-D-glucose and beta-D-galactose. Beta-D-galactose is isomerized into D-galactose. D-Galactose undergoes phosphorylation through a galactokinase, hence producing galactose 1 phosphate. On the other side of the pathway, a gluose-1-phosphate (product of the interaction of alpha-D-glucose 6-phosphate with a phosphoglucomutase resulting in a alpha-D-glucose-1-phosphate, an isomer of Glucose 1-phosphate, or an isomer of Beta-D-glucose 1-phosphate) interacts with UTP and a hydrogen ion in order to produce a uridine diphosphate glucose. This is followed by the interaction of galactose-1-phosphate with an established amount of uridine diphosphate glucose through a galactose-1-phosphate uridylyltransferase, which in turn output a glucose-1-phosphate and a uridine diphosphate galactose. The glucose -1-phosphate is transformed into a uridine diphosphate glucose through UTP--glucose-1-phosphate uridylyltransferase. The product, uridine diphosphate glucose, can undergo a reversible reaction in which it can be turned into uridine diphosphategalactose through an UDP-glucose 4-epimerase, and so the cycle can keep going as long as more lactose or galactose is imported into the cell
|
Metabolite
Metabolic
|
|
|
SMP0303611 |
Cardiolipin Biosynthesis CL(13:0/14:0/18:2(9Z,11Z)/a-21:0)Mus musculus
Cardiolipin (CL) is an important component of the inner mitochondrial membrane where it constitutes about 20% of the total lipid composition. It is essential for the optimal function of numerous enzymes that are involved in mitochondrial energy metabolism (Wikipedia). Cardiolipin biosynthesis occurs mainly in the mitochondria, but there also exists an alternative synthesis route for CDP-diacylglycerol that takes place in the endoplasmic reticulum. This second route may supplement this pathway. All membrane-localized enzymes are coloured dark green in the image. First, dihydroxyacetone phosphate (or glycerone phosphate) from glycolysis is used by the cytosolic enzyme glycerol-3-phosphate dehydrogenase [NAD(+)] to synthesize sn-glycerol 3-phosphate. Second, the mitochondrial outer membrane enzyme glycerol-3-phosphate acyltransferase esterifies an acyl-group to the sn-1 position of sn-glycerol 3-phosphate to form 1-acyl-sn-glycerol 3-phosphate (lysophosphatidic acid or LPA). Third, the enzyme 1-acyl-sn-glycerol-3-phosphate acyltransferase converts LPA into phosphatidic acid (PA or 1,2-diacyl-sn-glycerol 3-phosphate) by esterifying an acyl-group to the sn-2 position of the glycerol backbone. PA is then transferred to the inner mitochondrial membrane to continue cardiolipin synthesis. Fourth, magnesium-dependent phosphatidate cytidylyltransferase catalyzes the conversion of PA into CDP-diacylglycerol. Fifth, CDP-diacylglycerol--glycerol-3-phosphate 3-phosphatidyltransferase synthesizes phosphatidylglycerophosphate (PGP). Sixth, phosphatidylglycerophosphatase and protein-tyrosine phosphatase dephosphorylates PGP to form phosphatidylglycerol (PG). Last, cardiolipin synthase catalyzes the synthesis of cardiolipin by transferring a phosphatidyl group from a second CDP-diacylglycerol to PG. It requires a divalent metal cation cofactor.
|
Metabolite
Metabolic
|
|
|
SMP0303616 |
Cardiolipin Biosynthesis CL(13:0/14:0/18:2(9Z,11Z)/i-22:0)Mus musculus
Cardiolipin (CL) is an important component of the inner mitochondrial membrane where it constitutes about 20% of the total lipid composition. It is essential for the optimal function of numerous enzymes that are involved in mitochondrial energy metabolism (Wikipedia). Cardiolipin biosynthesis occurs mainly in the mitochondria, but there also exists an alternative synthesis route for CDP-diacylglycerol that takes place in the endoplasmic reticulum. This second route may supplement this pathway. All membrane-localized enzymes are coloured dark green in the image. First, dihydroxyacetone phosphate (or glycerone phosphate) from glycolysis is used by the cytosolic enzyme glycerol-3-phosphate dehydrogenase [NAD(+)] to synthesize sn-glycerol 3-phosphate. Second, the mitochondrial outer membrane enzyme glycerol-3-phosphate acyltransferase esterifies an acyl-group to the sn-1 position of sn-glycerol 3-phosphate to form 1-acyl-sn-glycerol 3-phosphate (lysophosphatidic acid or LPA). Third, the enzyme 1-acyl-sn-glycerol-3-phosphate acyltransferase converts LPA into phosphatidic acid (PA or 1,2-diacyl-sn-glycerol 3-phosphate) by esterifying an acyl-group to the sn-2 position of the glycerol backbone. PA is then transferred to the inner mitochondrial membrane to continue cardiolipin synthesis. Fourth, magnesium-dependent phosphatidate cytidylyltransferase catalyzes the conversion of PA into CDP-diacylglycerol. Fifth, CDP-diacylglycerol--glycerol-3-phosphate 3-phosphatidyltransferase synthesizes phosphatidylglycerophosphate (PGP). Sixth, phosphatidylglycerophosphatase and protein-tyrosine phosphatase dephosphorylates PGP to form phosphatidylglycerol (PG). Last, cardiolipin synthase catalyzes the synthesis of cardiolipin by transferring a phosphatidyl group from a second CDP-diacylglycerol to PG. It requires a divalent metal cation cofactor.
|
Metabolite
Metabolic
|
|
|
SMP0412506![]() |
Cardiolipin Biosynthesis CL(a-13:0/a-15:0/i-15:0/a-25:0)[rac]Mus musculus
Cardiolipin (CL) is an important component of the inner mitochondrial membrane where it constitutes about 20% of the total lipid composition. It is essential for the optimal function of numerous enzymes that are involved in mitochondrial energy metabolism (Wikipedia). Cardiolipin biosynthesis occurs mainly in the mitochondria, but there also exists an alternative synthesis route for CDP-diacylglycerol that takes place in the endoplasmic reticulum. This second route may supplement this pathway. All membrane-localized enzymes are coloured dark green in the image. First, dihydroxyacetone phosphate (or glycerone phosphate) from glycolysis is used by the cytosolic enzyme glycerol-3-phosphate dehydrogenase [NAD(+)] to synthesize sn-glycerol 3-phosphate. Second, the mitochondrial outer membrane enzyme glycerol-3-phosphate acyltransferase esterifies an acyl-group to the sn-1 position of sn-glycerol 3-phosphate to form 1-acyl-sn-glycerol 3-phosphate (lysophosphatidic acid or LPA). Third, the enzyme 1-acyl-sn-glycerol-3-phosphate acyltransferase converts LPA into phosphatidic acid (PA or 1,2-diacyl-sn-glycerol 3-phosphate) by esterifying an acyl-group to the sn-2 position of the glycerol backbone. PA is then transferred to the inner mitochondrial membrane to continue cardiolipin synthesis. Fourth, magnesium-dependent phosphatidate cytidylyltransferase catalyzes the conversion of PA into CDP-diacylglycerol. Fifth, CDP-diacylglycerol--glycerol-3-phosphate 3-phosphatidyltransferase synthesizes phosphatidylglycerophosphate (PGP). Sixth, phosphatidylglycerophosphatase and protein-tyrosine phosphatase dephosphorylates PGP to form phosphatidylglycerol (PG). Last, cardiolipin synthase catalyzes the synthesis of cardiolipin by transferring a phosphatidyl group from a second CDP-diacylglycerol to PG. It requires a divalent metal cation cofactor.
|
Metabolite
Metabolic
|
|
|
SMP0303724 |
Cardiolipin Biosynthesis CL(13:0/14:0/i-14:0/15:0)Mus musculus
Cardiolipin (CL) is an important component of the inner mitochondrial membrane where it constitutes about 20% of the total lipid composition. It is essential for the optimal function of numerous enzymes that are involved in mitochondrial energy metabolism (Wikipedia). Cardiolipin biosynthesis occurs mainly in the mitochondria, but there also exists an alternative synthesis route for CDP-diacylglycerol that takes place in the endoplasmic reticulum. This second route may supplement this pathway. All membrane-localized enzymes are coloured dark green in the image. First, dihydroxyacetone phosphate (or glycerone phosphate) from glycolysis is used by the cytosolic enzyme glycerol-3-phosphate dehydrogenase [NAD(+)] to synthesize sn-glycerol 3-phosphate. Second, the mitochondrial outer membrane enzyme glycerol-3-phosphate acyltransferase esterifies an acyl-group to the sn-1 position of sn-glycerol 3-phosphate to form 1-acyl-sn-glycerol 3-phosphate (lysophosphatidic acid or LPA). Third, the enzyme 1-acyl-sn-glycerol-3-phosphate acyltransferase converts LPA into phosphatidic acid (PA or 1,2-diacyl-sn-glycerol 3-phosphate) by esterifying an acyl-group to the sn-2 position of the glycerol backbone. PA is then transferred to the inner mitochondrial membrane to continue cardiolipin synthesis. Fourth, magnesium-dependent phosphatidate cytidylyltransferase catalyzes the conversion of PA into CDP-diacylglycerol. Fifth, CDP-diacylglycerol--glycerol-3-phosphate 3-phosphatidyltransferase synthesizes phosphatidylglycerophosphate (PGP). Sixth, phosphatidylglycerophosphatase and protein-tyrosine phosphatase dephosphorylates PGP to form phosphatidylglycerol (PG). Last, cardiolipin synthase catalyzes the synthesis of cardiolipin by transferring a phosphatidyl group from a second CDP-diacylglycerol to PG. It requires a divalent metal cation cofactor.
|
Metabolite
Metabolic
|
|
|
SMP0412698![]() |
Glycolate and Glyoxylate DegradationEscherichia coli IAI1
Glycolic acid is introduced into the cytoplasm through either a glycolate / lactate:H+ symporter or a acetate / glycolate transporter. Once inside, glycolic acid reacts with an oxidized electron-transfer flavoprotein through a glycolate oxidase resulting in a reduced acceptor and glyoxylic acid. Glyoxylic acid can also be obtained from the introduction of glyoxylic acid. It can also be obtained from the metabolism of (S)-allantoin.
S-allantoin is introduced into the cytoplasm through a purine and pyrimidine transporter(allantoin specific). Once inside, the compound reacts with water through a allantoinase resulting in hydrogen ion and allantoic acid. Allantoic acid then reacts with water and hydrogen ion through a allantoate amidohydrolase resulting in a carbon dioxide, ammonium and S-ureidoglycine. The latter compound reacts with water through a S-ureidoglycine aminohydrolase resulting in ammonium and S-ureidoglycolic acid which in turn reacts with a Ureidoglycolate lyase resulting in urea and glyoxylic acid.
Glyoxylic acid can either be metabolized into L-malic acid by a reaction with acetyl-CoA and Water through a malate synthase G which also releases hydrogen ion and Coenzyme A. L-malic acid is then incorporated into the TCA cycle.
Glyoxylic acid can also be metabolized by glyoxylate carboligase, releasing a carbon dioxide and tartronate semialdehyde. The latter compound is then reduced by an NADH driven tartronate semialdehyde reductase 2 resulting in glyceric acid. Glyceric acid is phosphorylated by a glycerate kinase 2 resulting in a 3-phosphoglyceric acid. This compound is then integrated into various other pathways: cysteine biosynthesis, serine biosynthesis and glycolysis and pyruvate dehydrogenase.
|
Metabolite
Metabolic
|
|
|
Showing 355041 -
355050 of 356704 pathways