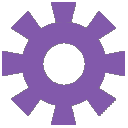
Browsing Pathways
Showing 355461 -
355470 of 605359 pathways
PathBank ID | Pathway Name and Description | Pathway Class | Chemical Compounds | Proteins |
---|---|---|---|---|
SMP0299551 |
Cardiolipin Biosynthesis CL(14:0/22:6(4Z,7Z,10Z,13Z,16Z,19Z)/22:6(4Z,7Z,10Z,13Z,16Z,19Z)/20:5(5Z,8Z,11Z,14Z,17Z))Mus musculus
Cardiolipin (CL) is an important component of the inner mitochondrial membrane where it constitutes about 20% of the total lipid composition. It is essential for the optimal function of numerous enzymes that are involved in mitochondrial energy metabolism (Wikipedia). Cardiolipin biosynthesis occurs mainly in the mitochondria, but there also exists an alternative synthesis route for CDP-diacylglycerol that takes place in the endoplasmic reticulum. This second route may supplement this pathway. All membrane-localized enzymes are coloured dark green in the image. First, dihydroxyacetone phosphate (or glycerone phosphate) from glycolysis is used by the cytosolic enzyme glycerol-3-phosphate dehydrogenase [NAD(+)] to synthesize sn-glycerol 3-phosphate. Second, the mitochondrial outer membrane enzyme glycerol-3-phosphate acyltransferase esterifies an acyl-group to the sn-1 position of sn-glycerol 3-phosphate to form 1-acyl-sn-glycerol 3-phosphate (lysophosphatidic acid or LPA). Third, the enzyme 1-acyl-sn-glycerol-3-phosphate acyltransferase converts LPA into phosphatidic acid (PA or 1,2-diacyl-sn-glycerol 3-phosphate) by esterifying an acyl-group to the sn-2 position of the glycerol backbone. PA is then transferred to the inner mitochondrial membrane to continue cardiolipin synthesis. Fourth, magnesium-dependent phosphatidate cytidylyltransferase catalyzes the conversion of PA into CDP-diacylglycerol. Fifth, CDP-diacylglycerol--glycerol-3-phosphate 3-phosphatidyltransferase synthesizes phosphatidylglycerophosphate (PGP). Sixth, phosphatidylglycerophosphatase and protein-tyrosine phosphatase dephosphorylates PGP to form phosphatidylglycerol (PG). Last, cardiolipin synthase catalyzes the synthesis of cardiolipin by transferring a phosphatidyl group from a second CDP-diacylglycerol to PG. It requires a divalent metal cation cofactor.
|
Metabolite
Metabolic
|
|
|
SMP0407247![]() |
Cardiolipin Biosynthesis CL(i-12:0/18:2(9Z,11Z)/a-25:0/i-24:0)[rac]Mus musculus
Cardiolipin (CL) is an important component of the inner mitochondrial membrane where it constitutes about 20% of the total lipid composition. It is essential for the optimal function of numerous enzymes that are involved in mitochondrial energy metabolism (Wikipedia). Cardiolipin biosynthesis occurs mainly in the mitochondria, but there also exists an alternative synthesis route for CDP-diacylglycerol that takes place in the endoplasmic reticulum. This second route may supplement this pathway. All membrane-localized enzymes are coloured dark green in the image. First, dihydroxyacetone phosphate (or glycerone phosphate) from glycolysis is used by the cytosolic enzyme glycerol-3-phosphate dehydrogenase [NAD(+)] to synthesize sn-glycerol 3-phosphate. Second, the mitochondrial outer membrane enzyme glycerol-3-phosphate acyltransferase esterifies an acyl-group to the sn-1 position of sn-glycerol 3-phosphate to form 1-acyl-sn-glycerol 3-phosphate (lysophosphatidic acid or LPA). Third, the enzyme 1-acyl-sn-glycerol-3-phosphate acyltransferase converts LPA into phosphatidic acid (PA or 1,2-diacyl-sn-glycerol 3-phosphate) by esterifying an acyl-group to the sn-2 position of the glycerol backbone. PA is then transferred to the inner mitochondrial membrane to continue cardiolipin synthesis. Fourth, magnesium-dependent phosphatidate cytidylyltransferase catalyzes the conversion of PA into CDP-diacylglycerol. Fifth, CDP-diacylglycerol--glycerol-3-phosphate 3-phosphatidyltransferase synthesizes phosphatidylglycerophosphate (PGP). Sixth, phosphatidylglycerophosphatase and protein-tyrosine phosphatase dephosphorylates PGP to form phosphatidylglycerol (PG). Last, cardiolipin synthase catalyzes the synthesis of cardiolipin by transferring a phosphatidyl group from a second CDP-diacylglycerol to PG. It requires a divalent metal cation cofactor.
|
Metabolite
Metabolic
|
|
|
SMP0398849![]() |
Gluconeogenesis from L-Malic AcidFusobacterium nucleatum subsp. vincentii 3_1_27
Gluconeogenesis from L-malic acid starts from the introduction of L-malic acid into cytoplasm either through a C4 dicarboxylate / orotate:H+ symporter or a dicarboxylate transporter (succinic acid antiporter). L-malic acid is then metabolized through 3 possible ways: NAD driven malate dehydrogenase resulting in oxalacetic acid, NADP driven malate dehydrogenase B resulting pyruvic acid or malate dehydrogenase, NAD-requiring resulting in pyruvic acid.
Oxalacetic acid is processed by phosphoenolpyruvate carboxykinase (ATP driven) while pyruvic acid is processed by phosphoenolpyruvate synthetase resulting in phosphoenolpyruvic acid. This compound is dehydrated by enolase resulting in an 2-phosphoglyceric acid which is then isomerized by 2,3-bisphosphoglycerate-independent phosphoglycerate mutase resulting in a 3-phosphoglyceric acid which is phosphorylated by an ATP driven phosphoglycerate kinase resulting in a glyceric acid 1,3-biphosphate. This compound undergoes an NADH driven glyceraldehyde 3-phosphate dehydrogenase reaction resulting in a D-Glyceraldehyde 3-phosphate which is first isomerized into dihydroxyacetone phosphate through an triosephosphate isomerase. D-glyceraldehyde 3-phosphate and Dihydroxyacetone phosphate react through a fructose biphosphate aldolase protein complex resulting in a fructose 1,6-biphosphate. Fructose 1,6-biphosphateis is metabolized by a fructose-1,6-bisphosphatase resulting in a Beta-D-fructofuranose 6-phosphate which is then isomerized into a Beta-D-glucose 6-phosphate through a glucose-6-phosphate isomerase.
|
Metabolite
Metabolic
|
|
|
SMP0398881![]() |
Tryptophan MetabolismFusobacterium periodonticum 2_1_31
The biosynthesis of L-tryptophan begins with L-glutamine interacting with a chorismate through a anthranilate synthase which results in a L-glutamic acid, a pyruvic acid, a hydrogen ion and a 2-aminobenzoic acid. The aminobenzoic acid interacts with a phosphoribosyl pyrophosphate through an anthranilate synthase component II resulting in a pyrophosphate and a N-(5-phosphoribosyl)-anthranilate. The latter compound is then metabolized by an indole-3-glycerol phosphate synthase / phosphoribosylanthranilate isomerase resulting in a 1-(o-carboxyphenylamino)-1-deoxyribulose 5'-phosphate. This compound then interacts with a hydrogen ion through a indole-3-glycerol phosphate synthase / phosphoribosylanthranilate isomerase resulting in the release of carbon dioxide, a water molecule and a (1S,2R)-1-C-(indol-3-yl)glycerol 3-phosphate. The latter compound then interacts with a D-glyceraldehyde 3-phosphate and an Indole. The indole interacts with an L-serine through a tryptophan synthase, β subunit dimer resulting in a water molecule and an L-tryptophan.
The metabolism of L-tryptophan starts with L-tryptophan being dehydrogenated by a tryptophanase / L-cysteine desulfhydrase resulting in the release of a hydrogen ion, an Indole and a 2-aminoacrylic acid. The latter compound is isomerized into a 2-iminopropanoate. This compound then interacts with a water molecule and a hydrogen ion spontaneously resulting in the release of an Ammonium and a pyruvic acid. The pyruvic acid then interacts with a coenzyme A through a NAD driven pyruvate dehydrogenase complex resulting in the release of a NADH, a carbon dioxide and an Acetyl-CoA
|
Metabolite
Metabolic
|
|
|
SMP0298019 |
Cardiolipin Biosynthesis CL(18:4(6Z,9Z,12Z,15Z)/18:2(9Z,12Z)/20:2(11Z,14Z)/16:1(9Z))Mus musculus
Cardiolipin (CL) is an important component of the inner mitochondrial membrane where it constitutes about 20% of the total lipid composition. It is essential for the optimal function of numerous enzymes that are involved in mitochondrial energy metabolism (Wikipedia). Cardiolipin biosynthesis occurs mainly in the mitochondria, but there also exists an alternative synthesis route for CDP-diacylglycerol that takes place in the endoplasmic reticulum. This second route may supplement this pathway. All membrane-localized enzymes are coloured dark green in the image. First, dihydroxyacetone phosphate (or glycerone phosphate) from glycolysis is used by the cytosolic enzyme glycerol-3-phosphate dehydrogenase [NAD(+)] to synthesize sn-glycerol 3-phosphate. Second, the mitochondrial outer membrane enzyme glycerol-3-phosphate acyltransferase esterifies an acyl-group to the sn-1 position of sn-glycerol 3-phosphate to form 1-acyl-sn-glycerol 3-phosphate (lysophosphatidic acid or LPA). Third, the enzyme 1-acyl-sn-glycerol-3-phosphate acyltransferase converts LPA into phosphatidic acid (PA or 1,2-diacyl-sn-glycerol 3-phosphate) by esterifying an acyl-group to the sn-2 position of the glycerol backbone. PA is then transferred to the inner mitochondrial membrane to continue cardiolipin synthesis. Fourth, magnesium-dependent phosphatidate cytidylyltransferase catalyzes the conversion of PA into CDP-diacylglycerol. Fifth, CDP-diacylglycerol--glycerol-3-phosphate 3-phosphatidyltransferase synthesizes phosphatidylglycerophosphate (PGP). Sixth, phosphatidylglycerophosphatase and protein-tyrosine phosphatase dephosphorylates PGP to form phosphatidylglycerol (PG). Last, cardiolipin synthase catalyzes the synthesis of cardiolipin by transferring a phosphatidyl group from a second CDP-diacylglycerol to PG. It requires a divalent metal cation cofactor.
|
Metabolite
Metabolic
|
|
|
SMP0394529![]() |
Histidine BiosynthesisBrevundimonas diminuta 470-4
Histidine biosynthesis starts with a product of PRPP biosynthesis pathway, phosphoribosyl pyrophosphate which interacts with a hydrogen ion through an ATP phosphoribosyltransferase resulting in an pyrophosphate and a phosphoribosyl-ATP. The phosphoribosyl-ATP interacts with water through a phosphoribosyl-AMP cyclohydrolase / phosphoribosyl-ATP pyrophosphatase resulting in the release of pyrophosphate, hydrogen ion and a phosphoribosyl-AMP. The same enzyme proceeds to interact with phosphoribosyl-AMP and water resulting in a 1-(5'-Phosphoribosyl)-5-amino-4-imidazolecarboxamide. The product is then isomerized by a N-(5'-phospho-L-ribosyl-formimino)-5-amino-1-(5'-phosphoribosyl)-4-imidazolecarboxamide isomerase resulting in a PhosphoribosylformiminoAICAR-phosphate, which reacts with L-glutamine through an imidazole glycerol phosphate synthase resulting in a L-glutamic acid, hydrogen ion, 5-aminoimidazole-4-carboxamide and a D-erythro-imidazole-glycerol-phosphate. D-erythro-imidazole-glycerol-phosphate reacts with a imidazoleglycerol-phosphate dehydratase / histidinol-phosphatase, dehydrating the compound and resulting in a imidazole acetol-phosphate. Next, imidazole acetol-phosphate reacts with L-glutamic acid through a histidinol-phosphate aminotransferase, releasing oxoglutaric acid and L-histidinol-phosphate. The latter compound interacts with water and a imidazoleglycerol-phosphate dehydratase / histidinol-phosphatase resulting in L-histidinol and phosphate. L-histidinol interacts with a NAD-driven histidinol dehydrogenase resulting in a Histidinal. Histidinal in turn reacts with water in a NAD driven histidinal dehydrogenase resulting in L-Histidine.
L-Histidine then represses ATP phosphoribosyltransferase, regulation its own biosynthesis.
|
Metabolite
Metabolic
|
|
|
SMP0394538![]() |
Methionine BiosynthesisBrevundimonas diminuta 470-4
This pathway shows the biosynthesis of methionine, which is an energy-costly process. Lysine biosynthesis produces L-Aspartate-semialdehyde, which later on is catalyzed to L-homoserine by bifunctional aspartokinase (also named homoserine dehydrogenase) 1 and 2. Homoserine is then activated by O-succinylation to form O-succinyl-L-homoserine via homoserine O-succinyltransferase (metA). Combining with L-cysteine, O-succinyl-L-homoserine form L-cystathionine and succinic acid by cystathionine gamma-synthase (metB). Cleavage of L-cystathionine by cystathionine beta-lyase (metC) or Protein MalY(as ) generates two small molecules: homocysteine and 2-aminoprop-2-enoate. Methionine synthase(MetH) or 5-methyltetrahydropteroyltriglutamate--homocysteine methyltransferase(MetE) will catalyzehomocysteine to form the final product: methionine. In E.coli, MetH can only function with existence of cobalamin (Vitamin B12), which can be available in the guy; without cobalamin, MetE will not be repressed so that it will catalyze the methionine. Methionine can be transported out of cell (into periplasmic space) by leucine efflux transporter.
|
Metabolite
Metabolic
|
|
|
SMP0394519![]() |
Leucine BiosynthesisFusobacterium varium ATCC 27725
Leucine biosynthesis involves a five-step conversion process starting with the valine precursor 2-keto-isovalerate interacting with acetyl-CoA and water through a 2-isopropylmalate synthase resulting in Coenzyme A, hydrogen Ion and 2-isopropylmalic acid. The latter compound reacts with isopropylmalate isomerase which dehydrates the compound resulting in a Isopropylmaleate. This compound reacts with water through a isopropylmalate isomerase resulting in 3-isopropylmalate. This compound interacts with a NAD-driven D-malate / 3-isopropylmalate dehydrogenase results in 2-isopropyl-3-oxosuccinate. This compound interacts spontaneously with hydrogen resulting in the release of carbon dioxide and ketoleucine. Ketoleucine interacts in a reversible reaction with L-glutamic acid through a branched-chain amino-acid aminotransferase resulting in Oxoglutaric acid and L-leucine. L-leucine can then be exported outside the cytoplasm through a transporter: L-amino acid efflux transporter. In the final step, ketoleucine can be catalyzed to form L-leucine by branched-chain amino-acid aminotransferase (IlvE) and tyrosine aminotransferase (TryB). L-Glutamic acid can also be transformed into oxoglutaric acid by these two enzymes. Tyrosine aminotransferase can be suppressed by lecuine, and inhibited by 2-keto-isovarlerate and its end product, tyrosine. 2-ketoisocaproate can not be introduced if 2-keto-isovarlerate inhibit TyrB and IlvE is absent.
|
Metabolite
Metabolic
|
||
SMP0394531![]() |
Leucine BiosynthesisBrevundimonas diminuta 470-4
Leucine biosynthesis involves a five-step conversion process starting with the valine precursor 2-keto-isovalerate interacting with acetyl-CoA and water through a 2-isopropylmalate synthase resulting in Coenzyme A, hydrogen Ion and 2-isopropylmalic acid. The latter compound reacts with isopropylmalate isomerase which dehydrates the compound resulting in a Isopropylmaleate. This compound reacts with water through a isopropylmalate isomerase resulting in 3-isopropylmalate. This compound interacts with a NAD-driven D-malate / 3-isopropylmalate dehydrogenase results in 2-isopropyl-3-oxosuccinate. This compound interacts spontaneously with hydrogen resulting in the release of carbon dioxide and ketoleucine. Ketoleucine interacts in a reversible reaction with L-glutamic acid through a branched-chain amino-acid aminotransferase resulting in Oxoglutaric acid and L-leucine. L-leucine can then be exported outside the cytoplasm through a transporter: L-amino acid efflux transporter. In the final step, ketoleucine can be catalyzed to form L-leucine by branched-chain amino-acid aminotransferase (IlvE) and tyrosine aminotransferase (TryB). L-Glutamic acid can also be transformed into oxoglutaric acid by these two enzymes. Tyrosine aminotransferase can be suppressed by lecuine, and inhibited by 2-keto-isovarlerate and its end product, tyrosine. 2-ketoisocaproate can not be introduced if 2-keto-isovarlerate inhibit TyrB and IlvE is absent.
|
Metabolite
Metabolic
|
||
SMP0398924![]() |
Chorismate BiosynthesisBrevundimonas bacteroides DSM 4726
Chorismate is an intermediate in tyrosine, phenylalanine and tryptophan synthesis and a precursor for folic acid, ubiquinone, enterochelin and menaquinone. Three enzymes catalyze the first step in chorismate biosynthesis. Synthesis may be reduced by feedback inhibition of tyrosine, phenylalanine and tryptophan to the enzymes. The biosynthesis of chorismate starts with D-Erythrose-4-phosphate getting transformed into 3-deoxy-D-arabino-heptulosonate-7-phosphate through a phospho-2-dehydro-3-deoxyheptonate aldolase. This is followed by a 3-dehydroquinate synthase converting the 3-deoxy-D-arabino-heptulosonate-7-phosphate into a 3-dehydroquinate which in turn is conveted to 3-dehydroshikimate through a 3-dehydroquinate dehydratase. At this point 3-dehydroshikimate can be turned into Shikimic acid through 2 different reactions involving Quinate/shikimate dehydrogenase and shikimate dehydrogenase 2. Shikimic acid is phosphorylated by Shikimate kinase 2 into shikimate 3-phosphate. Shikimate 3- phophate and a phosphoenolpyruvic acid are then joined through a 3-phosphoshikimate 1-carboxyvinyltransferase to produce a 5-enoylpyruvyl-shikimate 3-phosphate while releasing a phosphate. This in turn produces our final product Chorismate through a chorismate synthase.
|
Metabolite
Metabolic
|
|
Showing 355461 -
355470 of 359750 pathways