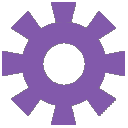
Browsing Pathways
Showing 365311 -
365320 of 605359 pathways
PathBank ID | Pathway Name and Description | Pathway Class | Chemical Compounds | Proteins |
---|---|---|---|---|
SMP0443867![]() |
Colanic Acid Building Blocks BiosynthesisBacteroides xylanisolvens XB1A
The colonic acid building blocks biosynthesis starts with a Beta-D-Glucose undergoing a transport reaction mediated by a glucose PTS permease. The permease phosphorylates the Beta-D-Glucose, producing a Beta-D-Glucose 6-phosphate. This compound can either change to an Alpha-D-Glucose 6-phosphate spontaneously or into a fructose 6-phosphate through a glucose-6-phosphate isomerase. The latter compound can also be present in E.coli through the interaction of D-fructose and a mannose PTS permease which phosphorylate the D-fructose.
Fructose 6-phosphate interacts in a reversible reaction with mannose-6-phosphate isomerase in order to produce a Alpha-D-mannose 6-phosphate. This compound can also be present in E.coli through the interaction of Alpha-D-mannose and a mannose PTS permease which phosphorylates the alpha-D-mannose. Alpha-D-mannose 6-phosphate interacts in a reversible reaction with a phosphomannomutase to produce a alpha-D-mannose 1-phosphate. This compound in turn with a hydrogen ion and gtp undergoes a reaction with a mannose-1-phosphate guanylyltransferase, releasing a pyrophosphate and producing a guanosine diphosphate mannose. Guanosine diphosphate mannose interacts with gdp-mannose 4,6-dehydratase releasing a water, and gdp-4-dehydro-6-deoxy-D-mannose. This compound in turn with hydrogen ion and NADPH interact with GDP-L-fucose synthase releasing NADP and producing a GDP-L-fucose.
The Alpha-D-Glucose 6-phosphate interacts in a reversible reaction with phosphoglucomutase-1 to produce a alpha-D-glucose 1-phosphate. This in turn with UTP and hydrogen ion interact with UTP--glucose-1-phosphate uridyleltransferase releasing a pyrophosphate and UDP-glucose.
UDP-glucose can either interact with galactose-1-phosphate uridylyltransferase to produce a UDP-galactose or in turn with NAD and water interact with UDP-glucose 6-dehydrogenase releasing a NADH and a hydrogen ion and producing a UDP-glucuronate.
GDP-L-fucose, UDP-glucose, UDP-galactose and UDP-glucuronate are sugars that need to be activated in the form of nucleotide sugar prior to their assembly into colanic acid, also known as M antigen.
Colanic acid is an extracellular polysaccharide which has been linked to a cluster of 19 genes(wca).
|
Metabolite
Metabolic
|
|
|
SMP0443862![]() |
Secondary Metabolites: Methylerythritol Phosphate and Polyisoprenoid BiosynthesisBacteroides thetaiotaomicron VPI-5482
The biosynthesis of isoprenoids starts with a D-glyceraldehyde 3-phosphate interacting with a hydrogen ion through a 1-deoxyxylulose-5-phosphate synthase resulting in a carbon dioxide and 1-Deoxy-D-xylulose. The latter compound then interacts with a hydrogen ion through a NADPH driven 1-deoxy-D-xylulose 5-phosphate reductoisomerase resulting in a NADP and a 2-C-methyl-D-erythritol 4-phosphate. The latter compound then interacts with a cytidine triphosphate and a hydrogen ion through a 4-diphosphocytidyl-2C-methyl-D-erythritol synthase resulting in a pyrophosphate and a 4-(cytidine 5'-diphospho)-2-C-methyl-D-erythritol. The latter compound is then phosphorylated through an ATP driven
4-diphosphocytidyl-2-C-methylerythritol kinase resulting in a release of an ADP, a hydrogen ion and a 2-phospho-4-(cytidine 5'-diphospho)-2-C-methyl-D-erythritol. The latter compound then interacts with a
2C-methyl-D-erythritol 2,4-cyclodiphosphate synthase resulting in the release of a 2-C-methyl-D-erythritol-2,4-cyclodiphosphate resulting in the release of a cytidine monophosphate and 2-C-methyl-D-erythritol-2,4-cyclodiphosphate. The latter compound then interacts with a reduced flavodoxin through a
1-hydroxy-2-methyl-2-(E)-butenyl 4-diphosphate synthase resulting in the release of a water molecule, a hydrogen ion, an oxidized flavodoxin and a 1-hydroxy-2-methyl-2-(E)-butenyl 4-diphosphate.
The compound 1-hydroxy-2-methyl-2-(E)-butenyl 4-diphosphate can interact with an NADPH,a hydrogen ion through a 1-hydroxy-2-methyl-2-(E)-butenyl 4-diphosphate reductase resulting in a NADP, a water molecule and either a Dimethylallylpyrophosphate or a Isopentenyl pyrophosphate. These two last compounds can be are isomers that can be produced through a isopentenyl diphosphate isomerase.
Dimethylallylpyrophosphate interacts with the isopentenyl pyrophosphate through a geranyl diphosphate synthase / farnesyl diphosphate synthase resulting in a pyrophosphate and a geranyl--PP. The latter compound interacts with a Isopentenyl pyrophosphate through a geranyl diphosphate synthase / farnesyl diphosphate synthase resulting in the release of a pyrophosphate and a farnesyl pyrophosphate. The latter compound interacts with isopentenyl pyrophosphate either through a undecaprenyl diphosphate synthase resulting in a release of a pyrophosphate and a di-trans,octa-cis-undecaprenyl diphosphate or through a octaprenyl diphosphate synthase resulting in a pyrophosphate and an octaprenyl diphosphate
|
Metabolite
Metabolic
|
|
|
SMP0338853 |
Cardiolipin Biosynthesis CL(a-13:0/21:0/i-24:0/i-24:0)Mus musculus
Cardiolipin (CL) is an important component of the inner mitochondrial membrane where it constitutes about 20% of the total lipid composition. It is essential for the optimal function of numerous enzymes that are involved in mitochondrial energy metabolism (Wikipedia). Cardiolipin biosynthesis occurs mainly in the mitochondria, but there also exists an alternative synthesis route for CDP-diacylglycerol that takes place in the endoplasmic reticulum. This second route may supplement this pathway. All membrane-localized enzymes are coloured dark green in the image. First, dihydroxyacetone phosphate (or glycerone phosphate) from glycolysis is used by the cytosolic enzyme glycerol-3-phosphate dehydrogenase [NAD(+)] to synthesize sn-glycerol 3-phosphate. Second, the mitochondrial outer membrane enzyme glycerol-3-phosphate acyltransferase esterifies an acyl-group to the sn-1 position of sn-glycerol 3-phosphate to form 1-acyl-sn-glycerol 3-phosphate (lysophosphatidic acid or LPA). Third, the enzyme 1-acyl-sn-glycerol-3-phosphate acyltransferase converts LPA into phosphatidic acid (PA or 1,2-diacyl-sn-glycerol 3-phosphate) by esterifying an acyl-group to the sn-2 position of the glycerol backbone. PA is then transferred to the inner mitochondrial membrane to continue cardiolipin synthesis. Fourth, magnesium-dependent phosphatidate cytidylyltransferase catalyzes the conversion of PA into CDP-diacylglycerol. Fifth, CDP-diacylglycerol--glycerol-3-phosphate 3-phosphatidyltransferase synthesizes phosphatidylglycerophosphate (PGP). Sixth, phosphatidylglycerophosphatase and protein-tyrosine phosphatase dephosphorylates PGP to form phosphatidylglycerol (PG). Last, cardiolipin synthase catalyzes the synthesis of cardiolipin by transferring a phosphatidyl group from a second CDP-diacylglycerol to PG. It requires a divalent metal cation cofactor.
|
Metabolite
Metabolic
|
|
|
SMP0338851 |
Cardiolipin Biosynthesis CL(a-13:0/21:0/i-24:0/25:0)Mus musculus
Cardiolipin (CL) is an important component of the inner mitochondrial membrane where it constitutes about 20% of the total lipid composition. It is essential for the optimal function of numerous enzymes that are involved in mitochondrial energy metabolism (Wikipedia). Cardiolipin biosynthesis occurs mainly in the mitochondria, but there also exists an alternative synthesis route for CDP-diacylglycerol that takes place in the endoplasmic reticulum. This second route may supplement this pathway. All membrane-localized enzymes are coloured dark green in the image. First, dihydroxyacetone phosphate (or glycerone phosphate) from glycolysis is used by the cytosolic enzyme glycerol-3-phosphate dehydrogenase [NAD(+)] to synthesize sn-glycerol 3-phosphate. Second, the mitochondrial outer membrane enzyme glycerol-3-phosphate acyltransferase esterifies an acyl-group to the sn-1 position of sn-glycerol 3-phosphate to form 1-acyl-sn-glycerol 3-phosphate (lysophosphatidic acid or LPA). Third, the enzyme 1-acyl-sn-glycerol-3-phosphate acyltransferase converts LPA into phosphatidic acid (PA or 1,2-diacyl-sn-glycerol 3-phosphate) by esterifying an acyl-group to the sn-2 position of the glycerol backbone. PA is then transferred to the inner mitochondrial membrane to continue cardiolipin synthesis. Fourth, magnesium-dependent phosphatidate cytidylyltransferase catalyzes the conversion of PA into CDP-diacylglycerol. Fifth, CDP-diacylglycerol--glycerol-3-phosphate 3-phosphatidyltransferase synthesizes phosphatidylglycerophosphate (PGP). Sixth, phosphatidylglycerophosphatase and protein-tyrosine phosphatase dephosphorylates PGP to form phosphatidylglycerol (PG). Last, cardiolipin synthase catalyzes the synthesis of cardiolipin by transferring a phosphatidyl group from a second CDP-diacylglycerol to PG. It requires a divalent metal cation cofactor.
|
Metabolite
Metabolic
|
|
|
SMP0338858 |
Cardiolipin Biosynthesis CL(a-15:0/21:0/21:0/25:0)Mus musculus
Cardiolipin (CL) is an important component of the inner mitochondrial membrane where it constitutes about 20% of the total lipid composition. It is essential for the optimal function of numerous enzymes that are involved in mitochondrial energy metabolism (Wikipedia). Cardiolipin biosynthesis occurs mainly in the mitochondria, but there also exists an alternative synthesis route for CDP-diacylglycerol that takes place in the endoplasmic reticulum. This second route may supplement this pathway. All membrane-localized enzymes are coloured dark green in the image. First, dihydroxyacetone phosphate (or glycerone phosphate) from glycolysis is used by the cytosolic enzyme glycerol-3-phosphate dehydrogenase [NAD(+)] to synthesize sn-glycerol 3-phosphate. Second, the mitochondrial outer membrane enzyme glycerol-3-phosphate acyltransferase esterifies an acyl-group to the sn-1 position of sn-glycerol 3-phosphate to form 1-acyl-sn-glycerol 3-phosphate (lysophosphatidic acid or LPA). Third, the enzyme 1-acyl-sn-glycerol-3-phosphate acyltransferase converts LPA into phosphatidic acid (PA or 1,2-diacyl-sn-glycerol 3-phosphate) by esterifying an acyl-group to the sn-2 position of the glycerol backbone. PA is then transferred to the inner mitochondrial membrane to continue cardiolipin synthesis. Fourth, magnesium-dependent phosphatidate cytidylyltransferase catalyzes the conversion of PA into CDP-diacylglycerol. Fifth, CDP-diacylglycerol--glycerol-3-phosphate 3-phosphatidyltransferase synthesizes phosphatidylglycerophosphate (PGP). Sixth, phosphatidylglycerophosphatase and protein-tyrosine phosphatase dephosphorylates PGP to form phosphatidylglycerol (PG). Last, cardiolipin synthase catalyzes the synthesis of cardiolipin by transferring a phosphatidyl group from a second CDP-diacylglycerol to PG. It requires a divalent metal cation cofactor.
|
Metabolite
Metabolic
|
|
|
SMP0423711![]() |
Purine Nucleotides De Novo BiosynthesisOxalobacter formigenes HOxBLS
The biosynthesis of purine nucleotides is a complex process that begins with a phosphoribosyl pyrophosphate. This compound interacts with water and L-glutamine through a
amidophosphoribosyl transferase resulting in a pyrophosphate, L-glutamic acid and a 5-phosphoribosylamine. The latter compound proceeds to interact with a glycine through an ATP driven phosphoribosylamine-glycine ligase resulting in the addition of glycine to the compound. This reaction releases an ADP, a phosphate, a hydrogen ion and a N1-(5-phospho-β-D-ribosyl)glycinamide. The latter compound interacts with formic acid, through an ATP driven phosphoribosylglycinamide formyltransferase 2 resulting in a phosphate, an ADP, a hydrogen ion and a 5-phosphoribosyl-N-formylglycinamide. The latter compound interacts with L-glutamine, and water through an ATP-driven
phosphoribosylformylglycinamide synthetase resulting in a release of a phosphate, an ADP, a hydrogen ion, a L-glutamic acid and a 2-(formamido)-N1-(5-phospho-D-ribosyl)acetamidine. The latter compound interacts with an ATP driven phosphoribosylformylglycinamide cyclo-ligase resulting in a release of ADP, a phosphate, a hydrogen ion and a 5-aminoimidazole ribonucleotide. The latter compound interacts with a hydrogen carbonate through an ATP driven N5-carboxyaminoimidazole ribonucleotide synthetase resulting in a release of a phosphate, an ADP, a hydrogen ion and a N5-carboxyaminoimidazole ribonucleotide.The latter compound then interacts with a N5-carboxyaminoimidazole ribonucleotide mutase resulting in a 5-amino-1-(5-phospho-D-ribosyl)imidazole-4-carboxylate. This compound interacts with an L-aspartic acid through an ATP driven phosphoribosylaminoimidazole-succinocarboxamide synthase resulting in a phosphate, an ADP, a hydrogen ion and a SAICAR. SAICAR interacts with an adenylosuccinate lyase resulting in a fumaric acid and an AICAR. AICAR interacts with a formyltetrahydrofolate through a AICAR transformylase / IMP cyclohydrolase resulting in a release of a tetrahydropterol mono-l-glutamate and a FAICAR. The latter compound, FAICAR, interacts in a reversible reaction through a AICAR transformylase / IMP cyclohydrolase resulting in a release of water and Inosinic acid.
Inosinic acid can be metabolized to produce dGTP and dATP three different methods each.
dGTP:
Inosinic acid, water and NAD are processed by IMP dehydrogenase resulting in a release of NADH, a hydrogen ion and Xanthylic acid. Xanthylic acid interacts with L-glutamine, and water through an ATP driven GMP synthetase resulting in pyrophosphate, AMP, L-glutamic acid, a hydrogen ion and Guanosine monophosphate. The latter compound is the phosphorylated by reacting with an ATP driven guanylate kinase resulting in a release of ADP and a Gaunosine diphosphate. Guanosine diphosphate can be metabolized in three different ways:
1.-Guanosine diphosphate is phosphorylated by an ATP-driven nucleoside diphosphate kinase resulting in an ADP and a Guanosine triphosphate. This compound interacts with a reduced flavodoxin protein through a ribonucleoside-triphosphate reductase resulting in a oxidized flavodoxin a water moleculer and a dGTP
2.-Guanosine diphosphate interacts with a reduced NrdH glutaredoxin-like proteins through a ribonucleoside-diphosphate reductase 2 resulting in the release of an oxidized NrdH glutaredoxin-like protein, a water molecule and a dGDP. The dGDP is then phosphorylated by interacting with an ATP-driven nucleoside diphosphate kinase resulting in an ADP and dGTP.
3.-Guanosine diphosphate interacts with a reduced thioredoxin ribonucleoside diphosphate reductase 1 resulting in a release of a water molecule, an oxidized thioredoxin and a dGDP. The dGDP is then phosphorylated by interacting with an ATP-driven nucleoside diphosphate kinase resulting in an ADP and dGTP.
dATP:
Inosinic acid interacts with L-aspartic acid through an GTP driven adenylosuccinate synthase results in the release of GDP, a hydrogen ion, a phosphate and N(6)-(1,2-dicarboxyethyl)AMP. The latter compound is then cleaved by a adenylosuccinate lyase resulting in a fumaric acid and an Adenosine monophosphate. This compound is then phosphorylated by an adenylate kinase resulting in the release of ATP and an adenosine diphosphate. Adenosine diphosphate can be metabolized in three different ways:
1.-Adenosine diphosphate is involved in a reversible reaction by interacting with a hydrogen ion and a phosphate through a ATP synthase / thiamin triphosphate synthase resulting in a hydrogen ion, a water molecule and an Adenosine triphosphate. The adenosine triphosphate interacts with a reduced flavodoxin through a ribonucleoside-triphosphate reductase resulting in an oxidized flavodoxin, a water molecule and a dATP
2.- Adenosine diphosphate interacts with an reduced thioredoxin through a ribonucleoside diphosphate reductase 1 resulting in a release of a water molecule, a oxidized thioredoxin and a dADP. The dADP is then phosphorylated by a nucleoside diphosphate kinase resulting in the release of ADP and a dATP
3.- Adenosine diphosphate interacts with an reduced NrdH glutaredoxin-like protein through a ribonucleoside diphosphate reductase 2 resulting in a release of a water molecule, a oxidized glutaredoxin-like protein and a dADP. The dADP is then phosphorylated by a nucleoside diphosphate kinase resulting in the release of ADP and a dATP
|
Metabolite
Metabolic
|
|
|
SMP0443945![]() |
Colanic Acid Building Blocks BiosynthesisCitrobacter freundii ATCC 8090 = MTCC 1658
The colonic acid building blocks biosynthesis starts with a Beta-D-Glucose undergoing a transport reaction mediated by a glucose PTS permease. The permease phosphorylates the Beta-D-Glucose, producing a Beta-D-Glucose 6-phosphate. This compound can either change to an Alpha-D-Glucose 6-phosphate spontaneously or into a fructose 6-phosphate through a glucose-6-phosphate isomerase. The latter compound can also be present in E.coli through the interaction of D-fructose and a mannose PTS permease which phosphorylate the D-fructose.
Fructose 6-phosphate interacts in a reversible reaction with mannose-6-phosphate isomerase in order to produce a Alpha-D-mannose 6-phosphate. This compound can also be present in E.coli through the interaction of Alpha-D-mannose and a mannose PTS permease which phosphorylates the alpha-D-mannose. Alpha-D-mannose 6-phosphate interacts in a reversible reaction with a phosphomannomutase to produce a alpha-D-mannose 1-phosphate. This compound in turn with a hydrogen ion and gtp undergoes a reaction with a mannose-1-phosphate guanylyltransferase, releasing a pyrophosphate and producing a guanosine diphosphate mannose. Guanosine diphosphate mannose interacts with gdp-mannose 4,6-dehydratase releasing a water, and gdp-4-dehydro-6-deoxy-D-mannose. This compound in turn with hydrogen ion and NADPH interact with GDP-L-fucose synthase releasing NADP and producing a GDP-L-fucose.
The Alpha-D-Glucose 6-phosphate interacts in a reversible reaction with phosphoglucomutase-1 to produce a alpha-D-glucose 1-phosphate. This in turn with UTP and hydrogen ion interact with UTP--glucose-1-phosphate uridyleltransferase releasing a pyrophosphate and UDP-glucose.
UDP-glucose can either interact with galactose-1-phosphate uridylyltransferase to produce a UDP-galactose or in turn with NAD and water interact with UDP-glucose 6-dehydrogenase releasing a NADH and a hydrogen ion and producing a UDP-glucuronate.
GDP-L-fucose, UDP-glucose, UDP-galactose and UDP-glucuronate are sugars that need to be activated in the form of nucleotide sugar prior to their assembly into colanic acid, also known as M antigen.
Colanic acid is an extracellular polysaccharide which has been linked to a cluster of 19 genes(wca).
|
Metabolite
Metabolic
|
|
|
SMP0443959![]() |
Secondary Metabolites: Threonine Biosynthesis from AspartateCampylobacter fetus subsp. fetus 82-40
The biosynthesis of threonine starts with L-aspartic acid being phosphorylated by an ATP driven Aspartate kinase resulting in an a release of an ADP and an L-aspartyl-4-phosphate. This compound interacts with a hydrogen ion through an NADPH driven aspartate semialdehyde dehydrogenase resulting in the release of a phosphate, an NADP and a L-aspartate-semialdehyde.The latter compound interacts with a hydrogen ion through a NADPH driven aspartate kinase / homoserine dehydrogenase resulting in the release of an NADP and a L-homoserine. L-homoserine is phosphorylated through an ATP driven homoserine kinase resulting in the release of an ADP, a hydrogen ion and a O-phosphohomoserine. The latter compound then interacts with a water molecule threonine synthase resulting in the release of a phosphate and an L-threonine.
|
Metabolite
Metabolic
|
||
SMP0443955![]() |
Secondary Metabolites: Enterobacterial Common Antigen BiosynthesisCitrobacter freundii ATCC 8090 = MTCC 1658
The biosynthesis of a enterobacterial common antigen can begin with a di-trans,octa-cis-undecaprenyl phosphate interacts with a Uridine diphosphate-N-acetylglucosamine through undecaprenyl-phosphate α-N-acetylglucosaminyl transferase resulting in a N-acetyl-α-D-glucosaminyl-diphospho-ditrans,octacis-undecaprenol and a Uridine 5'-monophosphate. The N-acetyl-α-D-glucosaminyl-diphospho-ditrans,octacis-undecaprenol then reacts with an UDP-ManNAcA from the Amino sugar and nucleotide sugar metabolism pathway. This reaction is metabolized by a UDP-N-acetyl-D-mannosaminuronic acid transferase resulting in a uridine 5' diphosphate, a hydrogen ion and a Undecaprenyl N-acetyl-glucosaminyl-N-acetyl-mannosaminuronate-4-acetamido-4,6-dideoxy-D-galactose pyrophosphate. Glucose 1 phosphate can be metabolize by interacting with a hydrogen ion and a thymidine 5-triphosphate by either reacting with a dTDP-glucose pyrophosphorylase or a dTDP-glucose pyrophosphorylase 2 resulting in the release of a pyrophosphate and a dTDP-D-glucose. The latter compound is then dehydrated through an dTDP-glucose 4,6-dehydratase 2 resulting in water and dTDP-4-dehydro-6-deoxy-D-glucose. The latter compound interacts with L-glutamic acid through a dTDP-4-dehydro-6-deoxy-D-glucose transaminase resulting in the release of oxoglutaric acid and dTDP-thomosamine. The latter compound interacts with acetyl-coa through a dTDP-fucosamine acetyltransferase resulting in a Coenzyme A, a hydrogen Ion and a TDP-Fuc4NAc. Undecaprenyl N-acetyl-glucosaminyl-N-acetyl-mannosaminuronate-4-acetamido-4,6-dideoxy-D-galactose pyrophosphate then interacts with a TDP--Fuc4NAc through a 4-acetamido-4,6-dideoxy-D-galactose transferase resulting in a hydrogen ion, a dTDP and a Undecaprenyl N-acetyl-glucosaminyl-N-acetyl-mannosaminuronate-4-acetamido-4,6-dideoxy-D-galactose pyrophosphate. This compound is then transported through a protein wzxE into the periplasmic space so that it can be incorporated into the outer membrane.
|
Metabolite
Metabolic
|
|
|
SMP0339048 |
Cardiolipin Biosynthesis CL(i-12:0/21:0/22:0/a-25:0)Mus musculus
Cardiolipin (CL) is an important component of the inner mitochondrial membrane where it constitutes about 20% of the total lipid composition. It is essential for the optimal function of numerous enzymes that are involved in mitochondrial energy metabolism (Wikipedia). Cardiolipin biosynthesis occurs mainly in the mitochondria, but there also exists an alternative synthesis route for CDP-diacylglycerol that takes place in the endoplasmic reticulum. This second route may supplement this pathway. All membrane-localized enzymes are coloured dark green in the image. First, dihydroxyacetone phosphate (or glycerone phosphate) from glycolysis is used by the cytosolic enzyme glycerol-3-phosphate dehydrogenase [NAD(+)] to synthesize sn-glycerol 3-phosphate. Second, the mitochondrial outer membrane enzyme glycerol-3-phosphate acyltransferase esterifies an acyl-group to the sn-1 position of sn-glycerol 3-phosphate to form 1-acyl-sn-glycerol 3-phosphate (lysophosphatidic acid or LPA). Third, the enzyme 1-acyl-sn-glycerol-3-phosphate acyltransferase converts LPA into phosphatidic acid (PA or 1,2-diacyl-sn-glycerol 3-phosphate) by esterifying an acyl-group to the sn-2 position of the glycerol backbone. PA is then transferred to the inner mitochondrial membrane to continue cardiolipin synthesis. Fourth, magnesium-dependent phosphatidate cytidylyltransferase catalyzes the conversion of PA into CDP-diacylglycerol. Fifth, CDP-diacylglycerol--glycerol-3-phosphate 3-phosphatidyltransferase synthesizes phosphatidylglycerophosphate (PGP). Sixth, phosphatidylglycerophosphatase and protein-tyrosine phosphatase dephosphorylates PGP to form phosphatidylglycerol (PG). Last, cardiolipin synthase catalyzes the synthesis of cardiolipin by transferring a phosphatidyl group from a second CDP-diacylglycerol to PG. It requires a divalent metal cation cofactor.
|
Metabolite
Metabolic
|
|
Showing 365311 -
365320 of 370498 pathways