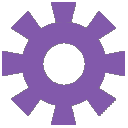
Browsing Pathways
Showing 366901 -
366910 of 605359 pathways
PathBank ID | Pathway Name and Description | Pathway Class | Chemical Compounds | Proteins |
---|---|---|---|---|
SMP0436492![]() |
PRPP BiosynthesisEscherichia coli IAI39
The biosynthesis of phosphoribosyl pyrophosphate begins as a product of the pentose phosphate and D-ribose 5-phosphate interaction. When catalyzed with a phosphopentomutase, the product is a ribose 1-phosphate. Ribose 1-phosphate can interact spontaneously with ATP resulting in a release of hydrogen ion, ADP and a ribose 1,5-biphosphate. Ribose 1,5-biphosphate is then phosphorylated through a ribose 1,5-bisphosphokinase resulting in the release of ADP and phosphoribosyl pyrophosphate. Phosphoribosyl pyrophosphate will then participate in the purine nucleotides de novo biosynthesis pathway. Alternatively pentose phosphate and D-ribose 5-phosphate's interaction can be phosphorylated through an ATP driven ribose-phosphate diphosphokinase resulting in a release of a hydrogen ion, an AMP and a phosphoribosyl pyrophosphate which will again participate in the purine nucleotides de novo biosynthesis pathway.
|
Metabolite
Metabolic
|
||
SMP0436508![]() |
Glutathione MetabolismHaemophilus influenzae PittEE
The biosynthesis of glutathione starts with the introduction of L-glutamic acid through either a glutamate:sodium symporter, glutamate / aspartate : H+ symporter GltP or a
glutamate / aspartate ABC transporter. Once in the cytoplasm, L-glutamice acid reacts with L-cysteine through an ATP glutamate-cysteine ligase resulting in gamma-glutamylcysteine. This compound reacts which Glycine through an ATP driven glutathione synthetase thus catabolizing Glutathione.
This compound is metabolized through a spontaneous reaction with an oxidized glutaredoxin resulting in a reduced glutaredoxin and an oxidized glutathione. This compound is reduced by a NADPH glutathione reductase resulting in a glutathione.
Glutathione can then be degraded into various different glutathione containing compounds by reacting with a napthalene or Bromobenzene-2,3-oxide through a glutathione S-transferase.
|
Metabolite
Metabolic
|
|
|
SMP0436466![]() |
Amino Sugar and Nucleotide Sugar Metabolism IIEscherichia coli O26:H11 str. 11368
The synthesis of amino sugars and nucleotide sugars starts with the phosphorylation of N-Acetylmuramic acid (MurNac) through its transport from the periplasmic space to the cytoplasm. Once in the cytoplasm, MurNac and water undergo a reversible reaction through a N-acetylmuramic acid 6-phosphate etherase, producing a D-lactic acid and N-Acetyl-D-Glucosamine 6-phosphate. This latter compound can also be introduced into the cytoplasm through a phosphorylating PTS permase in the inner membrane that allows for the transport of N-Acetyl-D-glucosamine from the periplasmic space. N-Acetyl-D-Glucosamine 6-phosphate can also be obtained from chitin dependent reactions. Chitin is hydrated through a bifunctional chitinase to produce chitobiose. This in turn gets hydrated by a beta-hexosaminidase to produce N-acetyl-D-glucosamine. The latter undergoes an atp dependent phosphorylation leading to the production of N-Acetyl-D-Glucosamine 6-phosphate.
N-Acetyl-D-Glucosamine 6-phosphate is then be deacetylated in order to produce Glucosamine 6-phosphate through a N-acetylglucosamine-6-phosphate deacetylase. This compound can either be isomerized or deaminated into Beta-D-fructofuranose 6-phosphate through a glucosamine-fructose-6-phosphate aminotransferase and a glucosamine-6-phosphate deaminase respectively.
Glucosamine 6-phosphate undergoes a reversible reaction to glucosamine 1 phosphate through a phosphoglucosamine mutase. This compound is then acetylated through a bifunctional protein glmU to produce a N-Acetyl glucosamine 1-phosphate.
N-Acetyl glucosamine 1-phosphate enters the nucleotide sugar synthesis by reacting with UTP and hydrogen ion through a bifunctional protein glmU releasing pyrophosphate and a Uridine diphosphate-N-acetylglucosamine.This compound can either be isomerized into a UDP-N-acetyl-D-mannosamine or undergo a reaction with phosphoenolpyruvic acid through UDP-N-acetylglucosamine 1-carboxyvinyltransferase releasing a phosphate and a UDP-N-Acetyl-alpha-D-glucosamine-enolpyruvate.
UDP-N-acetyl-D-mannosamine undergoes a NAD dependent dehydrogenation through a UDP-N-acetyl-D-mannosamine dehydrogenase, releasing NADH, a hydrogen ion and a UDP-N-Acetyl-alpha-D-mannosaminuronate, This compound is then used in the production of enterobacterial common antigens.
UDP-N-Acetyl-alpha-D-glucosamine-enolpyruvate is reduced through a NADPH dependent UDP-N-acetylenolpyruvoylglucosamine reductase, releasing a NADP and a UDP-N-acetyl-alpha-D-muramate. This compound is involved in the D-glutamine and D-glutamate metabolism.
|
Metabolite
Metabolic
|
|
|
SMP0436504![]() |
PRPP BiosynthesisEscherichia coli O103:H2 str. 12009
The biosynthesis of phosphoribosyl pyrophosphate begins as a product of the pentose phosphate and D-ribose 5-phosphate interaction. When catalyzed with a phosphopentomutase, the product is a ribose 1-phosphate. Ribose 1-phosphate can interact spontaneously with ATP resulting in a release of hydrogen ion, ADP and a ribose 1,5-biphosphate. Ribose 1,5-biphosphate is then phosphorylated through a ribose 1,5-bisphosphokinase resulting in the release of ADP and phosphoribosyl pyrophosphate. Phosphoribosyl pyrophosphate will then participate in the purine nucleotides de novo biosynthesis pathway. Alternatively pentose phosphate and D-ribose 5-phosphate's interaction can be phosphorylated through an ATP driven ribose-phosphate diphosphokinase resulting in a release of a hydrogen ion, an AMP and a phosphoribosyl pyrophosphate which will again participate in the purine nucleotides de novo biosynthesis pathway.
|
Metabolite
Metabolic
|
||
SMP0436862![]() |
Amino Sugar and Nucleotide Sugar Metabolism IIMycoplasma pneumoniae M129
The synthesis of amino sugars and nucleotide sugars starts with the phosphorylation of N-Acetylmuramic acid (MurNac) through its transport from the periplasmic space to the cytoplasm. Once in the cytoplasm, MurNac and water undergo a reversible reaction through a N-acetylmuramic acid 6-phosphate etherase, producing a D-lactic acid and N-Acetyl-D-Glucosamine 6-phosphate. This latter compound can also be introduced into the cytoplasm through a phosphorylating PTS permase in the inner membrane that allows for the transport of N-Acetyl-D-glucosamine from the periplasmic space. N-Acetyl-D-Glucosamine 6-phosphate can also be obtained from chitin dependent reactions. Chitin is hydrated through a bifunctional chitinase to produce chitobiose. This in turn gets hydrated by a beta-hexosaminidase to produce N-acetyl-D-glucosamine. The latter undergoes an atp dependent phosphorylation leading to the production of N-Acetyl-D-Glucosamine 6-phosphate.
N-Acetyl-D-Glucosamine 6-phosphate is then be deacetylated in order to produce Glucosamine 6-phosphate through a N-acetylglucosamine-6-phosphate deacetylase. This compound can either be isomerized or deaminated into Beta-D-fructofuranose 6-phosphate through a glucosamine-fructose-6-phosphate aminotransferase and a glucosamine-6-phosphate deaminase respectively.
Glucosamine 6-phosphate undergoes a reversible reaction to glucosamine 1 phosphate through a phosphoglucosamine mutase. This compound is then acetylated through a bifunctional protein glmU to produce a N-Acetyl glucosamine 1-phosphate.
N-Acetyl glucosamine 1-phosphate enters the nucleotide sugar synthesis by reacting with UTP and hydrogen ion through a bifunctional protein glmU releasing pyrophosphate and a Uridine diphosphate-N-acetylglucosamine.This compound can either be isomerized into a UDP-N-acetyl-D-mannosamine or undergo a reaction with phosphoenolpyruvic acid through UDP-N-acetylglucosamine 1-carboxyvinyltransferase releasing a phosphate and a UDP-N-Acetyl-alpha-D-glucosamine-enolpyruvate.
UDP-N-acetyl-D-mannosamine undergoes a NAD dependent dehydrogenation through a UDP-N-acetyl-D-mannosamine dehydrogenase, releasing NADH, a hydrogen ion and a UDP-N-Acetyl-alpha-D-mannosaminuronate, This compound is then used in the production of enterobacterial common antigens.
UDP-N-Acetyl-alpha-D-glucosamine-enolpyruvate is reduced through a NADPH dependent UDP-N-acetylenolpyruvoylglucosamine reductase, releasing a NADP and a UDP-N-acetyl-alpha-D-muramate. This compound is involved in the D-glutamine and D-glutamate metabolism.
|
Metabolite
Metabolic
|
|
|
SMP0436860![]() |
Amino Sugar and Nucleotide Sugar Metabolism IINeisseria gonorrhoeae FA 1090
The synthesis of amino sugars and nucleotide sugars starts with the phosphorylation of N-Acetylmuramic acid (MurNac) through its transport from the periplasmic space to the cytoplasm. Once in the cytoplasm, MurNac and water undergo a reversible reaction through a N-acetylmuramic acid 6-phosphate etherase, producing a D-lactic acid and N-Acetyl-D-Glucosamine 6-phosphate. This latter compound can also be introduced into the cytoplasm through a phosphorylating PTS permase in the inner membrane that allows for the transport of N-Acetyl-D-glucosamine from the periplasmic space. N-Acetyl-D-Glucosamine 6-phosphate can also be obtained from chitin dependent reactions. Chitin is hydrated through a bifunctional chitinase to produce chitobiose. This in turn gets hydrated by a beta-hexosaminidase to produce N-acetyl-D-glucosamine. The latter undergoes an atp dependent phosphorylation leading to the production of N-Acetyl-D-Glucosamine 6-phosphate.
N-Acetyl-D-Glucosamine 6-phosphate is then be deacetylated in order to produce Glucosamine 6-phosphate through a N-acetylglucosamine-6-phosphate deacetylase. This compound can either be isomerized or deaminated into Beta-D-fructofuranose 6-phosphate through a glucosamine-fructose-6-phosphate aminotransferase and a glucosamine-6-phosphate deaminase respectively.
Glucosamine 6-phosphate undergoes a reversible reaction to glucosamine 1 phosphate through a phosphoglucosamine mutase. This compound is then acetylated through a bifunctional protein glmU to produce a N-Acetyl glucosamine 1-phosphate.
N-Acetyl glucosamine 1-phosphate enters the nucleotide sugar synthesis by reacting with UTP and hydrogen ion through a bifunctional protein glmU releasing pyrophosphate and a Uridine diphosphate-N-acetylglucosamine.This compound can either be isomerized into a UDP-N-acetyl-D-mannosamine or undergo a reaction with phosphoenolpyruvic acid through UDP-N-acetylglucosamine 1-carboxyvinyltransferase releasing a phosphate and a UDP-N-Acetyl-alpha-D-glucosamine-enolpyruvate.
UDP-N-acetyl-D-mannosamine undergoes a NAD dependent dehydrogenation through a UDP-N-acetyl-D-mannosamine dehydrogenase, releasing NADH, a hydrogen ion and a UDP-N-Acetyl-alpha-D-mannosaminuronate, This compound is then used in the production of enterobacterial common antigens.
UDP-N-Acetyl-alpha-D-glucosamine-enolpyruvate is reduced through a NADPH dependent UDP-N-acetylenolpyruvoylglucosamine reductase, releasing a NADP and a UDP-N-acetyl-alpha-D-muramate. This compound is involved in the D-glutamine and D-glutamate metabolism.
|
Metabolite
Metabolic
|
|
|
SMP0436640![]() |
Porphyrin MetabolismAggregatibacter aphrophilus NJ8700
The metabolism of porphyrin begins with with glutamic acid being processed by an ATP-driven glutamyl-tRNA synthetase by interacting with hydrogen ion and tRNA(Glu), resulting in amo, pyrophosphate and L-glutamyl-tRNA(Glu) Glutamic acid. Glutamic acid can be obtained as a result of L-glutamate metabolism pathway, glutamate / aspartate : H+ symporter GltP, glutamate:sodium symporter or a glutamate / aspartate ABC transporter .
L-glutamyl-tRNA(Glu) Glutamic acid interacts with a NADPH glutamyl-tRNA reductase resulting in a NADP, a tRNA(Glu) and a (S)-4-amino-5-oxopentanoate.
This compound interacts with a glutamate-1-semialdehyde aminotransferase resulting a 5-aminolevulinic acid. This compound interacts with a porphobilinogen synthase resulting in a hydrogen ion, water and porphobilinogen. The latter compound interacts with water resulting in hydroxymethylbilane synthase resulting in ammonium, and hydroxymethylbilane.
Hydroxymethylbilane can either be dehydrated to produce uroporphyrinogen I or interact with a uroporphyrinogen III synthase resulting in a water molecule and a uroporphyrinogen III.
Uroporphyrinogen I interacts with hydrogen ion through a uroporphyrinogen decarboxylase resulting in a carbon dioxide and a coproporphyrinogen I
Uroporphyrinogen III can be metabolized into precorrin by interacting with a S-adenosylmethionine through a siroheme synthase resulting in hydrogen ion, an s-adenosylhomocysteine and a precorrin-1. On the other hand, Uroporphyrinogen III interacts with hydrogen ion through a uroporphyrinogen decarboxylase resulting in a carbon dioxide and a Coproporphyrinogen III.
Precorrin-1 reacts with a S-adenosylmethionine through a siroheme synthase resulting in a S-adenosylhomocysteine and a Precorrin-2. The latter compound is processed by a NAD dependent uroporphyrin III C-methyltransferase [multifunctional] resulting in a NADH and a sirohydrochlorin. This compound then interacts with Fe 2+
uroporphyrin III C-methyltransferase [multifunctional] resulting in a hydrogen ion and a siroheme. The siroheme is then processed in sulfur metabolism pathway.
Uroporphyrinogen III can be processed in anaerobic or aerobic condition.
Anaerobic:
Uroporphyrinogen III interacts with an oxygen molecule, a hydrogen ion through a coproporphyrinogen III oxidase resulting in water, carbon dioxide and protoporphyrinogen IX. The latter compound then interacts with an 3 oxygen molecule through a protoporphyrinogen oxidase resulting in 3 hydrogen peroxide and a Protoporphyrin IX
Aerobic:
Uroporphyrinogen III reacts with S-adenosylmethionine through a coproporphyrinogen III dehydrogenase resulting in carbon dioxide, 5-deoxyadenosine, L-methionine and protoporphyrinogen IX. The latter compound interacts with a meanquinone through a protoporphyrinogen oxidase resulting in protoporphyrin IX.
The protoporphyrin IX interacts with Fe 2+ through a ferrochelatase resulting in a hydrogen ion and a ferroheme b. The ferroheme b can either be incorporated into the oxidative phosphorylation as a cofactor of the enzymes involved in that pathway or it can interact with hydrogen peroxide through a catalase HPII resulting in a heme D. Heme D can then be incorporated into the oxidative phosphyrlation pathway as a cofactor of the enzymes involved in that pathway. Ferroheme b can also interact with water and a farnesyl pyrophosphate through a heme O synthase resulting in a release of pyrophosphate and heme O. Heme O is then incorporated into the Oxidative phosphorylation pathway.
|
Metabolite
Metabolic
|
|
|
SMP0436832![]() |
Amino Sugar and Nucleotide Sugar Metabolism IMoraxella catarrhalis RH4
The synthesis of amino sugars and nucleotide sugars starts with the phosphorylation of N-Acetylmuramic acid (MurNac) through its transport from the periplasmic space to the cytoplasm. Once in the cytoplasm, MurNac and water undergo a reversible reaction through a N-acetylmuramic acid 6-phosphate etherase, producing a D-lactic acid and N-Acetyl-D-Glucosamine 6-phosphate. This latter compound can also be introduced into the cytoplasm through a phosphorylating PTS permase in the inner membrane that allows for the transport of N-Acetyl-D-glucosamine from the periplasmic space. N-Acetyl-D-Glucosamine 6-phosphate can also be obtained from chitin dependent reactions. Chitin is hydrated through a bifunctional chitinase to produce chitobiose. This in turn gets hydrated by a beta-hexosaminidase to produce N-acetyl-D-glucosamine. The latter undergoes an atp dependent phosphorylation leading to the production of N-Acetyl-D-Glucosamine 6-phosphate.
N-Acetyl-D-Glucosamine 6-phosphate is then be deacetylated in order to produce Glucosamine 6-phosphate through a N-acetylglucosamine-6-phosphate deacetylase. This compound can either be isomerized or deaminated into Beta-D-fructofuranose 6-phosphate through a glucosamine-fructose-6-phosphate aminotransferase and a glucosamine-6-phosphate deaminase respectively.
Glucosamine 6-phosphate undergoes a reversible reaction to glucosamine 1 phosphate through a phosphoglucosamine mutase. This compound is then acetylated through a bifunctional protein glmU to produce a N-Acetyl glucosamine 1-phosphate.
N-Acetyl glucosamine 1-phosphate enters the nucleotide sugar synthesis by reacting with UTP and hydrogen ion through a bifunctional protein glmU releasing pyrophosphate and a Uridine diphosphate-N-acetylglucosamine.This compound can either be isomerized into a UDP-N-acetyl-D-mannosamine or undergo a reaction with phosphoenolpyruvic acid through UDP-N-acetylglucosamine 1-carboxyvinyltransferase releasing a phosphate and a UDP-N-Acetyl-alpha-D-glucosamine-enolpyruvate.
UDP-N-acetyl-D-mannosamine undergoes a NAD dependent dehydrogenation through a UDP-N-acetyl-D-mannosamine dehydrogenase, releasing NADH, a hydrogen ion and a UDP-N-Acetyl-alpha-D-mannosaminuronate, This compound is then used in the production of enterobacterial common antigens.
UDP-N-Acetyl-alpha-D-glucosamine-enolpyruvate is reduced through a NADPH dependent UDP-N-acetylenolpyruvoylglucosamine reductase, releasing a NADP and a UDP-N-acetyl-alpha-D-muramate. The latter is also involved in the D-glutamine and D-glutamate metabolism.
|
Metabolite
Metabolic
|
|
|
SMP0436826![]() |
Hexuronide and Hexuronate DegradationNeisseria meningitidis MC58
Beta-D-glucuronosides, D-glucuronate and D-fructuronate can be used as a source of carbon for E.coli. They are imported into E.coli's periplasmic space by membrane-associated protein (UidC/gusC), and are further imported into cytoplasm by hydrogen symporter. Beta-glucuronides undergoes hydrolysis by beta-D-glucuronidase to form D-glucuronate. D-glucuronate is isomerized by D-glucuronate isomerase to form D-fructuronate. D-fructuronate is further reduced to D-mannonate by D-mannonate oxidoreductase. D-mannonate dehydratase dehydrated to yield 2-dehydro-3-deoxy-D-gluconate. At this point, a common enzyme, 2-keto-3-deoxygluconokinase, phosphorylates 2-dehydro-3-deoxy-D-gluconate to yield 2-dehydro-3-deoxy-D-gluconate-6-phosphate. This product is then process by KHG/KDPG aldolase which in turn produces D-Glyceraldehyde 3-phosphate and Pyruvic Acid which then go into their respective sub pathways: glycolysis and pyruvate dehydrogenase. The pathway can also start from 3 other points: a hydrogen ion symporter (gluconate/fructuronate transporter GntP) of D-fructuronate, a hydrogen ion symporter (Hexuronate transporter) of aldehydo-D-galacturonate that spontaneously turns into D-tagaturonate. This compound can also be obtained by the reaction of aldehydo-L-galactonate with a NAD dependent l-galactonate oxidoreductase resulting in the release of NADH, hydrogen ion. Tagaturonate then undergoes an NADH-dependent reduction to D-altronate through an altronate oxidoreductase. D-altronate undergoes dehydration to yield 2-dehydro-3-deoxy-D-gluconate, the third and last point where the reaction can start from a hydrogen symporter of a 2-dehydro-3-deoy-D-gluconate.
|
Metabolite
Metabolic
|
|
|
SMP0436837![]() |
S-Adenosyl-L-Methionine BiosynthesisMycoplasma pneumoniae M129
S-adenosyl-L-methionine biosynthesis(SAM) is synthesized in the cytosol of the cell from L-methionine and ATP. This reaction is catalyzed by methionine adenosyltransferase. L methione is taken up from the environment through a complex reaction coupled transport and then proceeds too synthesize the s adenosylmethionine through a adenosylmethionine synthase. S-adenosylmethionine then interacts with a hydrogen ion through an adenosylmethionine decarboxylase resulting in a carbon dioxide and a S-adenosyl 3-methioninamine. This compound interacts with a putrescine through a spermidine synthase resulting in a spermidine, a hydrogen ion and a S-methyl-5'-thioadenosine. The latter compound is degraded by interacting with a water molecule through a 5' methylthioadenosine nucleosidase resulting in an adenine and a S-methylthioribose which is then release into the environment
|
Metabolite
Metabolic
|
|
Showing 366901 -
366910 of 367462 pathways