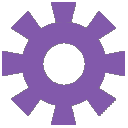
Browsing Pathways
Showing 367401 -
367410 of 605359 pathways
PathBank ID | Pathway Name and Description | Pathway Class | Chemical Compounds | Proteins |
---|---|---|---|---|
SMP0439891![]() |
Porphyrin MetabolismCampylobacter concisus 13826
The metabolism of porphyrin begins with with glutamic acid being processed by an ATP-driven glutamyl-tRNA synthetase by interacting with hydrogen ion and tRNA(Glu), resulting in amo, pyrophosphate and L-glutamyl-tRNA(Glu) Glutamic acid. Glutamic acid can be obtained as a result of L-glutamate metabolism pathway, glutamate / aspartate : H+ symporter GltP, glutamate:sodium symporter or a glutamate / aspartate ABC transporter .
L-glutamyl-tRNA(Glu) Glutamic acid interacts with a NADPH glutamyl-tRNA reductase resulting in a NADP, a tRNA(Glu) and a (S)-4-amino-5-oxopentanoate.
This compound interacts with a glutamate-1-semialdehyde aminotransferase resulting a 5-aminolevulinic acid. This compound interacts with a porphobilinogen synthase resulting in a hydrogen ion, water and porphobilinogen. The latter compound interacts with water resulting in hydroxymethylbilane synthase resulting in ammonium, and hydroxymethylbilane.
Hydroxymethylbilane can either be dehydrated to produce uroporphyrinogen I or interact with a uroporphyrinogen III synthase resulting in a water molecule and a uroporphyrinogen III.
Uroporphyrinogen I interacts with hydrogen ion through a uroporphyrinogen decarboxylase resulting in a carbon dioxide and a coproporphyrinogen I
Uroporphyrinogen III can be metabolized into precorrin by interacting with a S-adenosylmethionine through a siroheme synthase resulting in hydrogen ion, an s-adenosylhomocysteine and a precorrin-1. On the other hand, Uroporphyrinogen III interacts with hydrogen ion through a uroporphyrinogen decarboxylase resulting in a carbon dioxide and a Coproporphyrinogen III.
Precorrin-1 reacts with a S-adenosylmethionine through a siroheme synthase resulting in a S-adenosylhomocysteine and a Precorrin-2. The latter compound is processed by a NAD dependent uroporphyrin III C-methyltransferase [multifunctional] resulting in a NADH and a sirohydrochlorin. This compound then interacts with Fe 2+
uroporphyrin III C-methyltransferase [multifunctional] resulting in a hydrogen ion and a siroheme. The siroheme is then processed in sulfur metabolism pathway.
Uroporphyrinogen III can be processed in anaerobic or aerobic condition.
Anaerobic:
Uroporphyrinogen III interacts with an oxygen molecule, a hydrogen ion through a coproporphyrinogen III oxidase resulting in water, carbon dioxide and protoporphyrinogen IX. The latter compound then interacts with an 3 oxygen molecule through a protoporphyrinogen oxidase resulting in 3 hydrogen peroxide and a Protoporphyrin IX
Aerobic:
Uroporphyrinogen III reacts with S-adenosylmethionine through a coproporphyrinogen III dehydrogenase resulting in carbon dioxide, 5-deoxyadenosine, L-methionine and protoporphyrinogen IX. The latter compound interacts with a meanquinone through a protoporphyrinogen oxidase resulting in protoporphyrin IX.
The protoporphyrin IX interacts with Fe 2+ through a ferrochelatase resulting in a hydrogen ion and a ferroheme b. The ferroheme b can either be incorporated into the oxidative phosphorylation as a cofactor of the enzymes involved in that pathway or it can interact with hydrogen peroxide through a catalase HPII resulting in a heme D. Heme D can then be incorporated into the oxidative phosphyrlation pathway as a cofactor of the enzymes involved in that pathway. Ferroheme b can also interact with water and a farnesyl pyrophosphate through a heme O synthase resulting in a release of pyrophosphate and heme O. Heme O is then incorporated into the Oxidative phosphorylation pathway.
|
Metabolite
Metabolic
|
|
|
SMP0440498![]() |
PRPP BiosynthesisPseudomonas putida F1
The biosynthesis of phosphoribosyl pyrophosphate begins as a product of the pentose phosphate and D-ribose 5-phosphate interaction. When catalyzed with a phosphopentomutase, the product is a ribose 1-phosphate. Ribose 1-phosphate can interact spontaneously with ATP resulting in a release of hydrogen ion, ADP and a ribose 1,5-biphosphate. Ribose 1,5-biphosphate is then phosphorylated through a ribose 1,5-bisphosphokinase resulting in the release of ADP and phosphoribosyl pyrophosphate. Phosphoribosyl pyrophosphate will then participate in the purine nucleotides de novo biosynthesis pathway. Alternatively pentose phosphate and D-ribose 5-phosphate's interaction can be phosphorylated through an ATP driven ribose-phosphate diphosphokinase resulting in a release of a hydrogen ion, an AMP and a phosphoribosyl pyrophosphate which will again participate in the purine nucleotides de novo biosynthesis pathway.
|
Metabolite
Metabolic
|
||
SMP0440491![]() |
Folate BiosynthesisRhizobium leguminosarum bv. viciae 3841
The biosynthesis of folic acid begins as a product of purine nucleotides de novo biosynthesis pathway. Purine nucleotides are involved in a reaction with water through a GTP cyclohydrolase 1 protein complex, resulting in a hydrogen ion, formic acid and 7,8-dihydroneopterin 3-triphosphate. The latter compound is dephosphorylated through a dihydroneopterin triphosphate pyrophosphohydrolase resulting in the release of a pyrophosphate, hydrogen ion and 7,8-dihydroneopterin 3-phosphate. The latter product reacts with water spontaneously resulting in the release of a phosphate and a 7,8 -dihydroneopterin. 7,8 -dihydroneopterin reacts with a dihydroneopterin aldolase, releasing a glycoaldehyde and 6-hydroxymethyl-7,9-dihydropterin. Continuing, 6-hydroxymethyl-7,9-dihydropterin is phosphorylated with a ATP-driven 6-hydroxymethyl-7,8-dihydropterin pyrophosphokinase resulting in a (2-amino-4-hydroxy-7,8-dihydropteridin-6-yl)methyl diphosphate.
Chorismate is metabolized by reacting with L-glutamine through a 4-amino-4-deoxychorismate synthase resulting in L-glutamic acid and 4-amino-4-deoxychorismate. The latter product is then catalyzed via an aminodeoxychorismate lyase resulting in pyruvic acid, hydrogen ion and p-aminobenzoic acid.
(2-amino-4-hydroxy-7,8-dihydropteridin-6-yl)methyl diphosphate and p-aminobenzoic acid react with the help of a dihydropteroate synthase resulting in pyrophosphate and 7,8-dihydropteroic acid. This compound then reacts with L-glutamic acid through an ATP driven bifunctional folylpolyglutamate synthease / dihydrofolate synthease resulting in a 7,8-dihydrofolate monoglutamate. 7,8-dihydrofolate monoglutamate is then reduced via a NADPH mediated dihydrofolate reductase resulting in a tetrahydrofate which will continue and become a metabolite of the folate pathway
|
Metabolite
Metabolic
|
|
|
SMP0436871![]() |
Glutathione MetabolismSalmonella enterica subsp. enterica serovar Newport str. SL254
The biosynthesis of glutathione starts with the introduction of L-glutamic acid through either a glutamate:sodium symporter, glutamate / aspartate : H+ symporter GltP or a
glutamate / aspartate ABC transporter. Once in the cytoplasm, L-glutamice acid reacts with L-cysteine through an ATP glutamate-cysteine ligase resulting in gamma-glutamylcysteine. This compound reacts which Glycine through an ATP driven glutathione synthetase thus catabolizing Glutathione.
This compound is metabolized through a spontaneous reaction with an oxidized glutaredoxin resulting in a reduced glutaredoxin and an oxidized glutathione. This compound is reduced by a NADPH glutathione reductase resulting in a glutathione.
Glutathione can then be degraded into various different glutathione containing compounds by reacting with a napthalene or Bromobenzene-2,3-oxide through a glutathione S-transferase.
|
Metabolite
Metabolic
|
|
|
SMP0439898![]() |
Fructose MetabolismCampylobacter jejuni subsp. jejuni 81116
Fructose metabolism begins with the transport of Beta-D-fructofuranose through a fructose PTS permease, resulting in a Beta-D-fructofuranose 1-phosphate. This compound is phosphorylated by an ATP driven 1-phosphofructokinase resulting in a fructose 1,6-biphosphate. This compound can either react with a fructose bisphosphate aldolase class 1 resulting in D-glyceraldehyde 3-phosphate and a dihydroxyacetone phosphate or through a fructose biphosphate aldolase class 2 resulting in a D-glyceraldehyde 3-phosphate. This compound can then either react in a reversible triosephosphate isomerase resulting in a dihydroxyacetone phosphate or react with a phosphate through a NAD dependent Glyceraldehyde 3-phosphate dehydrogenase resulting in a glyceric acid 1,3-biphosphate. This compound is desphosphorylated by a phosphoglycerate kinase resulting in a 3-phosphoglyceric acid.This compound in turn can either react with a 2,3-bisphosphoglycerate-independent phosphoglycerate mutase or a 2,3-bisphosphoglycerate-independent phosphoglycerate mutase resulting in a 2-phospho-D-glyceric acid. This compound interacts with an enolase resulting in a phosphoenolpyruvic acid and water. Phosphoenolpyruvic acid can react either through a AMP driven phosphoenoylpyruvate synthase or a ADP driven pyruvate kinase protein complex resulting in a pyruvic acid.
Pyruvic acid reacts with CoA through a NAD driven pyruvate dehydrogenase complex resulting in a carbon dioxide and a Acetyl-CoA which gets incorporated into the TCA cycle pathway.
|
Metabolite
Metabolic
|
|
|
SMP0336110 |
Cardiolipin Biosynthesis CL(20:3(11Z,14Z,17Z)/20:4(5Z,8Z,11Z,14Z)/20:3(11Z,14Z,17Z)/22:5(4Z,7Z,10Z,13Z,16Z))Mus musculus
Cardiolipin (CL) is an important component of the inner mitochondrial membrane where it constitutes about 20% of the total lipid composition. It is essential for the optimal function of numerous enzymes that are involved in mitochondrial energy metabolism (Wikipedia). Cardiolipin biosynthesis occurs mainly in the mitochondria, but there also exists an alternative synthesis route for CDP-diacylglycerol that takes place in the endoplasmic reticulum. This second route may supplement this pathway. All membrane-localized enzymes are coloured dark green in the image. First, dihydroxyacetone phosphate (or glycerone phosphate) from glycolysis is used by the cytosolic enzyme glycerol-3-phosphate dehydrogenase [NAD(+)] to synthesize sn-glycerol 3-phosphate. Second, the mitochondrial outer membrane enzyme glycerol-3-phosphate acyltransferase esterifies an acyl-group to the sn-1 position of sn-glycerol 3-phosphate to form 1-acyl-sn-glycerol 3-phosphate (lysophosphatidic acid or LPA). Third, the enzyme 1-acyl-sn-glycerol-3-phosphate acyltransferase converts LPA into phosphatidic acid (PA or 1,2-diacyl-sn-glycerol 3-phosphate) by esterifying an acyl-group to the sn-2 position of the glycerol backbone. PA is then transferred to the inner mitochondrial membrane to continue cardiolipin synthesis. Fourth, magnesium-dependent phosphatidate cytidylyltransferase catalyzes the conversion of PA into CDP-diacylglycerol. Fifth, CDP-diacylglycerol--glycerol-3-phosphate 3-phosphatidyltransferase synthesizes phosphatidylglycerophosphate (PGP). Sixth, phosphatidylglycerophosphatase and protein-tyrosine phosphatase dephosphorylates PGP to form phosphatidylglycerol (PG). Last, cardiolipin synthase catalyzes the synthesis of cardiolipin by transferring a phosphatidyl group from a second CDP-diacylglycerol to PG. It requires a divalent metal cation cofactor.
|
Metabolite
Metabolic
|
|
|
SMP0436873![]() |
S-Adenosyl-L-Methionine BiosynthesisSalmonella enterica subsp. enterica serovar Heidelberg str. SL476
S-adenosyl-L-methionine biosynthesis(SAM) is synthesized in the cytosol of the cell from L-methionine and ATP. This reaction is catalyzed by methionine adenosyltransferase. L methione is taken up from the environment through a complex reaction coupled transport and then proceeds too synthesize the s adenosylmethionine through a adenosylmethionine synthase. S-adenosylmethionine then interacts with a hydrogen ion through an adenosylmethionine decarboxylase resulting in a carbon dioxide and a S-adenosyl 3-methioninamine. This compound interacts with a putrescine through a spermidine synthase resulting in a spermidine, a hydrogen ion and a S-methyl-5'-thioadenosine. The latter compound is degraded by interacting with a water molecule through a 5' methylthioadenosine nucleosidase resulting in an adenine and a S-methylthioribose which is then release into the environment
|
Metabolite
Metabolic
|
|
|
SMP0334917 |
Cardiolipin Biosynthesis CL(22:5(7Z,10Z,13Z,16Z,19Z)/20:3(11Z,14Z,17Z)/22:5(4Z,7Z,10Z,13Z,16Z)/22:5(4Z,7Z,10Z,13Z,16Z))Mus musculus
Cardiolipin (CL) is an important component of the inner mitochondrial membrane where it constitutes about 20% of the total lipid composition. It is essential for the optimal function of numerous enzymes that are involved in mitochondrial energy metabolism (Wikipedia). Cardiolipin biosynthesis occurs mainly in the mitochondria, but there also exists an alternative synthesis route for CDP-diacylglycerol that takes place in the endoplasmic reticulum. This second route may supplement this pathway. All membrane-localized enzymes are coloured dark green in the image. First, dihydroxyacetone phosphate (or glycerone phosphate) from glycolysis is used by the cytosolic enzyme glycerol-3-phosphate dehydrogenase [NAD(+)] to synthesize sn-glycerol 3-phosphate. Second, the mitochondrial outer membrane enzyme glycerol-3-phosphate acyltransferase esterifies an acyl-group to the sn-1 position of sn-glycerol 3-phosphate to form 1-acyl-sn-glycerol 3-phosphate (lysophosphatidic acid or LPA). Third, the enzyme 1-acyl-sn-glycerol-3-phosphate acyltransferase converts LPA into phosphatidic acid (PA or 1,2-diacyl-sn-glycerol 3-phosphate) by esterifying an acyl-group to the sn-2 position of the glycerol backbone. PA is then transferred to the inner mitochondrial membrane to continue cardiolipin synthesis. Fourth, magnesium-dependent phosphatidate cytidylyltransferase catalyzes the conversion of PA into CDP-diacylglycerol. Fifth, CDP-diacylglycerol--glycerol-3-phosphate 3-phosphatidyltransferase synthesizes phosphatidylglycerophosphate (PGP). Sixth, phosphatidylglycerophosphatase and protein-tyrosine phosphatase dephosphorylates PGP to form phosphatidylglycerol (PG). Last, cardiolipin synthase catalyzes the synthesis of cardiolipin by transferring a phosphatidyl group from a second CDP-diacylglycerol to PG. It requires a divalent metal cation cofactor.
|
Metabolite
Metabolic
|
|
|
SMP0334929 |
Cardiolipin Biosynthesis CL(22:5(7Z,10Z,13Z,16Z,19Z)/20:3(11Z,14Z,17Z)/22:5(7Z,10Z,13Z,16Z,19Z)/18:0)Mus musculus
Cardiolipin (CL) is an important component of the inner mitochondrial membrane where it constitutes about 20% of the total lipid composition. It is essential for the optimal function of numerous enzymes that are involved in mitochondrial energy metabolism (Wikipedia). Cardiolipin biosynthesis occurs mainly in the mitochondria, but there also exists an alternative synthesis route for CDP-diacylglycerol that takes place in the endoplasmic reticulum. This second route may supplement this pathway. All membrane-localized enzymes are coloured dark green in the image. First, dihydroxyacetone phosphate (or glycerone phosphate) from glycolysis is used by the cytosolic enzyme glycerol-3-phosphate dehydrogenase [NAD(+)] to synthesize sn-glycerol 3-phosphate. Second, the mitochondrial outer membrane enzyme glycerol-3-phosphate acyltransferase esterifies an acyl-group to the sn-1 position of sn-glycerol 3-phosphate to form 1-acyl-sn-glycerol 3-phosphate (lysophosphatidic acid or LPA). Third, the enzyme 1-acyl-sn-glycerol-3-phosphate acyltransferase converts LPA into phosphatidic acid (PA or 1,2-diacyl-sn-glycerol 3-phosphate) by esterifying an acyl-group to the sn-2 position of the glycerol backbone. PA is then transferred to the inner mitochondrial membrane to continue cardiolipin synthesis. Fourth, magnesium-dependent phosphatidate cytidylyltransferase catalyzes the conversion of PA into CDP-diacylglycerol. Fifth, CDP-diacylglycerol--glycerol-3-phosphate 3-phosphatidyltransferase synthesizes phosphatidylglycerophosphate (PGP). Sixth, phosphatidylglycerophosphatase and protein-tyrosine phosphatase dephosphorylates PGP to form phosphatidylglycerol (PG). Last, cardiolipin synthase catalyzes the synthesis of cardiolipin by transferring a phosphatidyl group from a second CDP-diacylglycerol to PG. It requires a divalent metal cation cofactor.
|
Metabolite
Metabolic
|
|
|
SMP0334934 |
Cardiolipin Biosynthesis CL(22:5(7Z,10Z,13Z,16Z,19Z)/20:3(11Z,14Z,17Z)/22:5(7Z,10Z,13Z,16Z,19Z)/18:4(6Z,9Z,12Z,15Z))Mus musculus
Cardiolipin (CL) is an important component of the inner mitochondrial membrane where it constitutes about 20% of the total lipid composition. It is essential for the optimal function of numerous enzymes that are involved in mitochondrial energy metabolism (Wikipedia). Cardiolipin biosynthesis occurs mainly in the mitochondria, but there also exists an alternative synthesis route for CDP-diacylglycerol that takes place in the endoplasmic reticulum. This second route may supplement this pathway. All membrane-localized enzymes are coloured dark green in the image. First, dihydroxyacetone phosphate (or glycerone phosphate) from glycolysis is used by the cytosolic enzyme glycerol-3-phosphate dehydrogenase [NAD(+)] to synthesize sn-glycerol 3-phosphate. Second, the mitochondrial outer membrane enzyme glycerol-3-phosphate acyltransferase esterifies an acyl-group to the sn-1 position of sn-glycerol 3-phosphate to form 1-acyl-sn-glycerol 3-phosphate (lysophosphatidic acid or LPA). Third, the enzyme 1-acyl-sn-glycerol-3-phosphate acyltransferase converts LPA into phosphatidic acid (PA or 1,2-diacyl-sn-glycerol 3-phosphate) by esterifying an acyl-group to the sn-2 position of the glycerol backbone. PA is then transferred to the inner mitochondrial membrane to continue cardiolipin synthesis. Fourth, magnesium-dependent phosphatidate cytidylyltransferase catalyzes the conversion of PA into CDP-diacylglycerol. Fifth, CDP-diacylglycerol--glycerol-3-phosphate 3-phosphatidyltransferase synthesizes phosphatidylglycerophosphate (PGP). Sixth, phosphatidylglycerophosphatase and protein-tyrosine phosphatase dephosphorylates PGP to form phosphatidylglycerol (PG). Last, cardiolipin synthase catalyzes the synthesis of cardiolipin by transferring a phosphatidyl group from a second CDP-diacylglycerol to PG. It requires a divalent metal cation cofactor.
|
Metabolite
Metabolic
|
|
Showing 367401 -
367410 of 368007 pathways