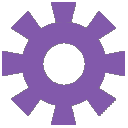
Browsing Pathways
Showing 367581 -
367590 of 605359 pathways
PathBank ID | Pathway Name and Description | Pathway Class | Chemical Compounds | Proteins |
---|---|---|---|---|
SMP0439651![]() |
Hexuronide and Hexuronate DegradationYersinia kristensenii ATCC 33638
Beta-D-glucuronosides, D-glucuronate and D-fructuronate can be used as a source of carbon for E.coli. They are imported into E.coli's periplasmic space by membrane-associated protein (UidC/gusC), and are further imported into cytoplasm by hydrogen symporter. Beta-glucuronides undergoes hydrolysis by beta-D-glucuronidase to form D-glucuronate. D-glucuronate is isomerized by D-glucuronate isomerase to form D-fructuronate. D-fructuronate is further reduced to D-mannonate by D-mannonate oxidoreductase. D-mannonate dehydratase dehydrated to yield 2-dehydro-3-deoxy-D-gluconate. At this point, a common enzyme, 2-keto-3-deoxygluconokinase, phosphorylates 2-dehydro-3-deoxy-D-gluconate to yield 2-dehydro-3-deoxy-D-gluconate-6-phosphate. This product is then process by KHG/KDPG aldolase which in turn produces D-Glyceraldehyde 3-phosphate and Pyruvic Acid which then go into their respective sub pathways: glycolysis and pyruvate dehydrogenase. The pathway can also start from 3 other points: a hydrogen ion symporter (gluconate/fructuronate transporter GntP) of D-fructuronate, a hydrogen ion symporter (Hexuronate transporter) of aldehydo-D-galacturonate that spontaneously turns into D-tagaturonate. This compound can also be obtained by the reaction of aldehydo-L-galactonate with a NAD dependent l-galactonate oxidoreductase resulting in the release of NADH, hydrogen ion. Tagaturonate then undergoes an NADH-dependent reduction to D-altronate through an altronate oxidoreductase. D-altronate undergoes dehydration to yield 2-dehydro-3-deoxy-D-gluconate, the third and last point where the reaction can start from a hydrogen symporter of a 2-dehydro-3-deoy-D-gluconate.
|
Metabolite
Metabolic
|
|
|
SMP0439677![]() |
Amino Sugar and Nucleotide Sugar Metabolism IYersinia bercovieri ATCC 43970
The synthesis of amino sugars and nucleotide sugars starts with the phosphorylation of N-Acetylmuramic acid (MurNac) through its transport from the periplasmic space to the cytoplasm. Once in the cytoplasm, MurNac and water undergo a reversible reaction through a N-acetylmuramic acid 6-phosphate etherase, producing a D-lactic acid and N-Acetyl-D-Glucosamine 6-phosphate. This latter compound can also be introduced into the cytoplasm through a phosphorylating PTS permase in the inner membrane that allows for the transport of N-Acetyl-D-glucosamine from the periplasmic space. N-Acetyl-D-Glucosamine 6-phosphate can also be obtained from chitin dependent reactions. Chitin is hydrated through a bifunctional chitinase to produce chitobiose. This in turn gets hydrated by a beta-hexosaminidase to produce N-acetyl-D-glucosamine. The latter undergoes an atp dependent phosphorylation leading to the production of N-Acetyl-D-Glucosamine 6-phosphate.
N-Acetyl-D-Glucosamine 6-phosphate is then be deacetylated in order to produce Glucosamine 6-phosphate through a N-acetylglucosamine-6-phosphate deacetylase. This compound can either be isomerized or deaminated into Beta-D-fructofuranose 6-phosphate through a glucosamine-fructose-6-phosphate aminotransferase and a glucosamine-6-phosphate deaminase respectively.
Glucosamine 6-phosphate undergoes a reversible reaction to glucosamine 1 phosphate through a phosphoglucosamine mutase. This compound is then acetylated through a bifunctional protein glmU to produce a N-Acetyl glucosamine 1-phosphate.
N-Acetyl glucosamine 1-phosphate enters the nucleotide sugar synthesis by reacting with UTP and hydrogen ion through a bifunctional protein glmU releasing pyrophosphate and a Uridine diphosphate-N-acetylglucosamine.This compound can either be isomerized into a UDP-N-acetyl-D-mannosamine or undergo a reaction with phosphoenolpyruvic acid through UDP-N-acetylglucosamine 1-carboxyvinyltransferase releasing a phosphate and a UDP-N-Acetyl-alpha-D-glucosamine-enolpyruvate.
UDP-N-acetyl-D-mannosamine undergoes a NAD dependent dehydrogenation through a UDP-N-acetyl-D-mannosamine dehydrogenase, releasing NADH, a hydrogen ion and a UDP-N-Acetyl-alpha-D-mannosaminuronate, This compound is then used in the production of enterobacterial common antigens.
UDP-N-Acetyl-alpha-D-glucosamine-enolpyruvate is reduced through a NADPH dependent UDP-N-acetylenolpyruvoylglucosamine reductase, releasing a NADP and a UDP-N-acetyl-alpha-D-muramate. The latter is also involved in the D-glutamine and D-glutamate metabolism.
|
Metabolite
Metabolic
|
|
|
SMP0439672![]() |
Amino Sugar and Nucleotide Sugar Metabolism IYersinia kristensenii ATCC 33638
The synthesis of amino sugars and nucleotide sugars starts with the phosphorylation of N-Acetylmuramic acid (MurNac) through its transport from the periplasmic space to the cytoplasm. Once in the cytoplasm, MurNac and water undergo a reversible reaction through a N-acetylmuramic acid 6-phosphate etherase, producing a D-lactic acid and N-Acetyl-D-Glucosamine 6-phosphate. This latter compound can also be introduced into the cytoplasm through a phosphorylating PTS permase in the inner membrane that allows for the transport of N-Acetyl-D-glucosamine from the periplasmic space. N-Acetyl-D-Glucosamine 6-phosphate can also be obtained from chitin dependent reactions. Chitin is hydrated through a bifunctional chitinase to produce chitobiose. This in turn gets hydrated by a beta-hexosaminidase to produce N-acetyl-D-glucosamine. The latter undergoes an atp dependent phosphorylation leading to the production of N-Acetyl-D-Glucosamine 6-phosphate.
N-Acetyl-D-Glucosamine 6-phosphate is then be deacetylated in order to produce Glucosamine 6-phosphate through a N-acetylglucosamine-6-phosphate deacetylase. This compound can either be isomerized or deaminated into Beta-D-fructofuranose 6-phosphate through a glucosamine-fructose-6-phosphate aminotransferase and a glucosamine-6-phosphate deaminase respectively.
Glucosamine 6-phosphate undergoes a reversible reaction to glucosamine 1 phosphate through a phosphoglucosamine mutase. This compound is then acetylated through a bifunctional protein glmU to produce a N-Acetyl glucosamine 1-phosphate.
N-Acetyl glucosamine 1-phosphate enters the nucleotide sugar synthesis by reacting with UTP and hydrogen ion through a bifunctional protein glmU releasing pyrophosphate and a Uridine diphosphate-N-acetylglucosamine.This compound can either be isomerized into a UDP-N-acetyl-D-mannosamine or undergo a reaction with phosphoenolpyruvic acid through UDP-N-acetylglucosamine 1-carboxyvinyltransferase releasing a phosphate and a UDP-N-Acetyl-alpha-D-glucosamine-enolpyruvate.
UDP-N-acetyl-D-mannosamine undergoes a NAD dependent dehydrogenation through a UDP-N-acetyl-D-mannosamine dehydrogenase, releasing NADH, a hydrogen ion and a UDP-N-Acetyl-alpha-D-mannosaminuronate, This compound is then used in the production of enterobacterial common antigens.
UDP-N-Acetyl-alpha-D-glucosamine-enolpyruvate is reduced through a NADPH dependent UDP-N-acetylenolpyruvoylglucosamine reductase, releasing a NADP and a UDP-N-acetyl-alpha-D-muramate. The latter is also involved in the D-glutamine and D-glutamate metabolism.
|
Metabolite
Metabolic
|
|
|
SMP0439740![]() |
Glutathione MetabolismNevskia ramosa DSM 11499
The biosynthesis of glutathione starts with the introduction of L-glutamic acid through either a glutamate:sodium symporter, glutamate / aspartate : H+ symporter GltP or a
glutamate / aspartate ABC transporter. Once in the cytoplasm, L-glutamice acid reacts with L-cysteine through an ATP glutamate-cysteine ligase resulting in gamma-glutamylcysteine. This compound reacts which Glycine through an ATP driven glutathione synthetase thus catabolizing Glutathione.
This compound is metabolized through a spontaneous reaction with an oxidized glutaredoxin resulting in a reduced glutaredoxin and an oxidized glutathione. This compound is reduced by a NADPH glutathione reductase resulting in a glutathione.
Glutathione can then be degraded into various different glutathione containing compounds by reacting with a napthalene or Bromobenzene-2,3-oxide through a glutathione S-transferase.
|
Metabolite
Metabolic
|
|
|
SMP0439694![]() |
Amino Sugar and Nucleotide Sugar Metabolism IIIYersinia kristensenii ATCC 33638
The synthesis of amino sugars and nucleotide sugars starts with the phosphorylation of N-Acetylmuramic acid (MurNac) through its transport from the periplasmic space to the cytoplasm. Once in the cytoplasm, MurNac and water undergo a reversible reaction catalyzed by N-acetylmuramic acid 6-phosphate etherase, producing a D-lactic acid and N-Acetyl-D-Glucosamine 6-phosphate. This latter compound can also be introduced into the cytoplasm through a phosphorylating PTS permase in the inner membrane that allows for the transport of N-Acetyl-D-glucosamine from the periplasmic space. N-Acetyl-D-Glucosamine 6-phosphate can also be obtained from chitin dependent reactions. Chitin is hydrated through a bifunctional chitinase to produce chitobiose. This in turn gets hydrated by a beta-hexosaminidase to produce N-acetyl-D-glucosamine. The latter undergoes an atp dependent phosphorylation leading to the production of N-Acetyl-D-Glucosamine 6-phosphate. N-Acetyl-D-Glucosamine 6-phosphate is then be deacetylated in order to produce Glucosamine 6-phosphate through a N-acetylglucosamine-6-phosphate deacetylase. This compound can either be isomerized or deaminated into Beta-D-fructofuranose 6-phosphate through a glucosamine-fructose-6-phosphate aminotransferase and a glucosamine-6-phosphate deaminase respectively.
Glucosamine 6-phosphate undergoes a reversible reaction to glucosamine 1 phosphate through a phosphoglucosamine mutase. This compound is then acetylated through a bifunctional protein glmU to produce a N-Acetyl glucosamine 1-phosphate.
N-Acetyl glucosamine 1-phosphate enters the nucleotide sugar synthesis by reacting with UTP and hydrogen ion through a bifunctional protein glmU releasing pyrophosphate and a Uridine diphosphate-N-acetylglucosamine.This compound can either be isomerized into a UDP-N-acetyl-D-mannosamine or undergo a reaction with phosphoenolpyruvic acid through UDP-N-acetylglucosamine 1-carboxyvinyltransferase releasing a phosphate and a UDP-N-Acetyl-alpha-D-glucosamine-enolpyruvate.
UDP-N-acetyl-D-mannosamine undergoes a NAD dependent dehydrogenation through a UDP-N-acetyl-D-mannosamine dehydrogenase, releasing NADH, a hydrogen ion and a UDP-N-Acetyl-alpha-D-mannosaminuronate, This compound is then used in the production of enterobacterial common antigens.
UDP-N-Acetyl-alpha-D-glucosamine-enolpyruvate is reduced through a NADPH dependent UDP-N-acetylenolpyruvoylglucosamine reductase, releasing a NADP and a UDP-N-acetyl-alpha-D-muramate. This compound is involved in the D-glutamine and D-glutamate metabolism.
|
Metabolite
Metabolic
|
|
|
SMP0439700![]() |
Fatty Acid BiosynthesisYersinia bercovieri ATCC 43970
The fatty acid biosynthesis starts from acetyl-CoA reacting either with a holo-[acp] through a 3-oxoacyl-[acp] synthase 3 resulting in an acetyl-[acp] or react with hydrogen carbonate through an ATP driven acetyl-CoA carboxylase resulting in a malonyl-CoA.
Malonyl-CoA reacts with a holo-acp] through a malonyl-CoA-ACP transacylase resulting in a malonyl-[acp]. This compound can react with a KASI protein resulting in an acetyl-[acp]. A malonyl-[acp] can also react with an acetyl-[acp] through KASI and KASII or with acetyl-CoA through a beta-ketoacyl-ACP synthase to produce an acetoacetyl-[acp]. An acetoacetyl-[acp] is also known as a 3-oxoacyl-[acp].
A 3-oxoacyl-[acp] is reduced through a NDPH mediated 3-oxoacyl-[acp] reductase resulting in a (3R)-3-hydroxyacyl-[acp] (R3 hydroxydecanoyl-[acp]) which can either join the fatty acid metabolism, be dehydrated by an 3R-hydroxymyristoyl-[acp] dehydratase to produce a trans-2-enoyl-[acp] or be dehydrated by a hydroxydecanoyl-[acp] to produce a trans-delta2 decenoyl-[acp].
Trans-2-enoyl-[acp] is reduced by a NADH driven enoyl-[acp] reductase resulting in a 2,3,4-saturated fatty acyl-[acp]. This product then reacts with malonyl-[acp] through KASI and KASII resulting in a holo-acyl carrier protein and a 3- oxoacyl-[acp].
Trans-delta2 decenoyl-[acp] reacts with a 3-hydroxydecanoyl-[acp] dehydrase producing a cis-delta 3-decenoyl-ACP. This product then reacts with KASI to produce a 3-oxo-cis-delta5-dodecenoyl-[acp], which in turn is reduced by a NADPH driven 3-oxoacyl-[acp] resulting in a 3R-hydroxy cis delta5-dodecenoyl-acp. This product is dehydrated by a (3R)-hydroxymyristoyl-[acp] dehydratase resulting in a trans-delta 3- cis-delta 5-dodecenoyl-[acp] which in turn is reduced by a NADH driven enoyl-[acp] reductase resulting in a cis-delta5-dodecenoyl-acp which becomes a metabolite of fatty acid metabolism
|
Metabolite
Metabolic
|
||
SMP0439717![]() |
Fatty Acid BiosynthesisYersinia kristensenii ATCC 33638
The fatty acid biosynthesis starts from acetyl-CoA reacting either with a holo-[acp] through a 3-oxoacyl-[acp] synthase 3 resulting in an acetyl-[acp] or react with hydrogen carbonate through an ATP driven acetyl-CoA carboxylase resulting in a malonyl-CoA.
Malonyl-CoA reacts with a holo-acp] through a malonyl-CoA-ACP transacylase resulting in a malonyl-[acp]. This compound can react with a KASI protein resulting in an acetyl-[acp]. A malonyl-[acp] can also react with an acetyl-[acp] through KASI and KASII or with acetyl-CoA through a beta-ketoacyl-ACP synthase to produce an acetoacetyl-[acp]. An acetoacetyl-[acp] is also known as a 3-oxoacyl-[acp].
A 3-oxoacyl-[acp] is reduced through a NDPH mediated 3-oxoacyl-[acp] reductase resulting in a (3R)-3-hydroxyacyl-[acp] (R3 hydroxydecanoyl-[acp]) which can either join the fatty acid metabolism, be dehydrated by an 3R-hydroxymyristoyl-[acp] dehydratase to produce a trans-2-enoyl-[acp] or be dehydrated by a hydroxydecanoyl-[acp] to produce a trans-delta2 decenoyl-[acp].
Trans-2-enoyl-[acp] is reduced by a NADH driven enoyl-[acp] reductase resulting in a 2,3,4-saturated fatty acyl-[acp]. This product then reacts with malonyl-[acp] through KASI and KASII resulting in a holo-acyl carrier protein and a 3- oxoacyl-[acp].
Trans-delta2 decenoyl-[acp] reacts with a 3-hydroxydecanoyl-[acp] dehydrase producing a cis-delta 3-decenoyl-ACP. This product then reacts with KASI to produce a 3-oxo-cis-delta5-dodecenoyl-[acp], which in turn is reduced by a NADPH driven 3-oxoacyl-[acp] resulting in a 3R-hydroxy cis delta5-dodecenoyl-acp. This product is dehydrated by a (3R)-hydroxymyristoyl-[acp] dehydratase resulting in a trans-delta 3- cis-delta 5-dodecenoyl-[acp] which in turn is reduced by a NADH driven enoyl-[acp] reductase resulting in a cis-delta5-dodecenoyl-acp which becomes a metabolite of fatty acid metabolism
|
Metabolite
Metabolic
|
||
SMP0439647![]() |
Glutathione MetabolismYersinia kristensenii ATCC 33638
The biosynthesis of glutathione starts with the introduction of L-glutamic acid through either a glutamate:sodium symporter, glutamate / aspartate : H+ symporter GltP or a
glutamate / aspartate ABC transporter. Once in the cytoplasm, L-glutamice acid reacts with L-cysteine through an ATP glutamate-cysteine ligase resulting in gamma-glutamylcysteine. This compound reacts which Glycine through an ATP driven glutathione synthetase thus catabolizing Glutathione.
This compound is metabolized through a spontaneous reaction with an oxidized glutaredoxin resulting in a reduced glutaredoxin and an oxidized glutathione. This compound is reduced by a NADPH glutathione reductase resulting in a glutathione.
Glutathione can then be degraded into various different glutathione containing compounds by reacting with a napthalene or Bromobenzene-2,3-oxide through a glutathione S-transferase.
|
Metabolite
Metabolic
|
|
|
SMP0439670![]() |
Galactose Degradation/Leloir PathwayYersinia bercovieri ATCC 43970
The degradation of galactose, also known as Leloir pathway, requires 3 main enzymes once Beta-D-galactose has been converted to galactose through an Aldose-1-epimerase. These are: galactokinase , galactose-1-phosphate uridylyltransferase and UDP-glucose 4-epimerase. Beta-D-galactose can be uptaken from the environment through a galactose proton symporter. It can also be produced by lactose degradation involving a lactose permease to uptake lactose from the environment and a beta-galactosidase to turn lactose into Beta-D-galactose.
Galactose is degraded through the following process:
Beta-D-galactose is introduced into the cytoplasm through a galactose proton symporter, or it can be synthesized from an alpha lactose that is introduced into the cytoplasm through a lactose permease. Alpha lactose interacts with water through a beta-galactosidase resulting in a beta-D-glucose and beta-D-galactose. Beta-D-galactose is isomerized into D-galactose. D-Galactose undergoes phosphorylation through a galactokinase, hence producing galactose 1 phosphate. On the other side of the pathway, a gluose-1-phosphate (product of the interaction of alpha-D-glucose 6-phosphate with a phosphoglucomutase resulting in a alpha-D-glucose-1-phosphate, an isomer of Glucose 1-phosphate, or an isomer of Beta-D-glucose 1-phosphate) interacts with UTP and a hydrogen ion in order to produce a uridine diphosphate glucose. This is followed by the interaction of galactose-1-phosphate with an established amount of uridine diphosphate glucose through a galactose-1-phosphate uridylyltransferase, which in turn output a glucose-1-phosphate and a uridine diphosphate galactose. The glucose -1-phosphate is transformed into a uridine diphosphate glucose through UTP--glucose-1-phosphate uridylyltransferase. The product, uridine diphosphate glucose, can undergo a reversible reaction in which it can be turned into uridine diphosphategalactose through an UDP-glucose 4-epimerase, and so the cycle can keep going as long as more lactose or galactose is imported into the cell.
|
Metabolite
Metabolic
|
|
|
SMP0440667![]() |
Sulfur Metabolism (Ethanesulfonate)Escherichia coli O157:H7 str. Sakai
The sulfur metabolism pathway starts in three possible ways. The first is the uptake of sulfate through an active transport reaction via a sulfate transport system containing an ATP-binding protein which hydrolyses ATP. Sulfate is converted by the sulfate adenylyltransferase enzymatic complex to adenosine phosphosulfate through the addition of adenine from a molecule of ATP, along with one phosphate group. Adenosine phosphosulfate is further converted to phoaphoadenosine phosphosulfate through an ATP hydrolysis and dehydrogenation reaction by the adenylyl-sulfate kinase. Phoaphoadenosine phosphosulfate is finally dehydrogenated and converted to sulfite by phosphoadenosine phosphosulfate reductase. This reaction requires magnesium, and adenosine 3',5'-diphosphate is the bi-product. A thioredoxin is also oxidized. Sulfite can also be produced from the dehydrogenation of cyanide along with the conversion of thiosulfate to thiocyanate by the thiosulfate sulfurtransferase enzymatic complex. Sulfite next undergoes a series of reactions that lead to the production of pyruvic acid, which is a precursor for pathways such as gluconeogenesis. The first reaction in this series is the conversion of sulfite to hydrogen sulfide through hygrogenation and the deoxygenation of sulfite to form a water molecule. The reaction is catalyzed by the sulfite reductase [NADPH] flavoprotein alpha and beta components. Siroheme, 4Fe-4S, flavin mononucleotide, and FAD function as cofactors or prosthetic groups. Hydrogen sulfide next undergoes dehydrogenation in a reversible reaction to form L-Cysteine and acetic acid, via the cysteine synthase complex and the coenzyme pyridoxal 5'-phosphate. L-Cysteine is dehydrogenated and converted to 2-aminoacrylic acid (a bronsted acid) and hydrogen sulfide(which may be reused) by a larger enzymatic complex composed of cysteine synthase A/B, protein malY, cystathionine-β-lyase, and tryptophanase, along with the coenzyme pyridoxal 5'-phosphate. 2-aminoacrylic acid isomerizes to 2-iminopropanoate, which along with a water molecule and a hydrogen ion is lastly converted to pyruvic acid and ammonium in a spontaneous fashion. The second possible initial starting point for sulfur metabolism is the import of taurine(an alternate sulfur source) into the cytoplasm via the taurine ABC transporter complex. Taurine, oxoglutaric acid, and oxygen are converted to sulfite by the alpha-ketoglutarate-dependent taurine dioxygenase. Carbon dioxide, succinic acid, and aminoacetaldehyde are bi-products of this reaction. Sulfite next enters pyruvic acid synthesis as already described. The third variant of sulfur metabolism starts with the import of an alkyl sulfate, in this case 1-butanesulfonate, into the cytoplasm via an aliphatic sulfonate ABC transporter complex which hydrolyses ATP. 1-butanesulfonate is dehydrogenated and along with oxygen is converted to sulfite and betaine aldehyde by the FMNH2-dependent alkanesulfonate monooxygenase enzyme. Water and flavin mononucleotide(which is used in a subsequent reaction as a prosthetic group) are also produced. Sulfite is next converted to pyruvic acid by the process already described.
|
Metabolite
Metabolic
|
|
|
Showing 367581 -
367590 of 368894 pathways