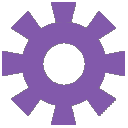
Browsing Pathways
Showing 367641 -
367650 of 605359 pathways
PathBank ID | Pathway Name and Description | Pathway Class | Chemical Compounds | Proteins |
---|---|---|---|---|
SMP0437210![]() |
Amino Sugar and Nucleotide Sugar Metabolism IIIBacteroides xylanisolvens SD CC 1b
The synthesis of amino sugars and nucleotide sugars starts with the phosphorylation of N-Acetylmuramic acid (MurNac) through its transport from the periplasmic space to the cytoplasm. Once in the cytoplasm, MurNac and water undergo a reversible reaction catalyzed by N-acetylmuramic acid 6-phosphate etherase, producing a D-lactic acid and N-Acetyl-D-Glucosamine 6-phosphate. This latter compound can also be introduced into the cytoplasm through a phosphorylating PTS permase in the inner membrane that allows for the transport of N-Acetyl-D-glucosamine from the periplasmic space. N-Acetyl-D-Glucosamine 6-phosphate can also be obtained from chitin dependent reactions. Chitin is hydrated through a bifunctional chitinase to produce chitobiose. This in turn gets hydrated by a beta-hexosaminidase to produce N-acetyl-D-glucosamine. The latter undergoes an atp dependent phosphorylation leading to the production of N-Acetyl-D-Glucosamine 6-phosphate. N-Acetyl-D-Glucosamine 6-phosphate is then be deacetylated in order to produce Glucosamine 6-phosphate through a N-acetylglucosamine-6-phosphate deacetylase. This compound can either be isomerized or deaminated into Beta-D-fructofuranose 6-phosphate through a glucosamine-fructose-6-phosphate aminotransferase and a glucosamine-6-phosphate deaminase respectively.
Glucosamine 6-phosphate undergoes a reversible reaction to glucosamine 1 phosphate through a phosphoglucosamine mutase. This compound is then acetylated through a bifunctional protein glmU to produce a N-Acetyl glucosamine 1-phosphate.
N-Acetyl glucosamine 1-phosphate enters the nucleotide sugar synthesis by reacting with UTP and hydrogen ion through a bifunctional protein glmU releasing pyrophosphate and a Uridine diphosphate-N-acetylglucosamine.This compound can either be isomerized into a UDP-N-acetyl-D-mannosamine or undergo a reaction with phosphoenolpyruvic acid through UDP-N-acetylglucosamine 1-carboxyvinyltransferase releasing a phosphate and a UDP-N-Acetyl-alpha-D-glucosamine-enolpyruvate.
UDP-N-acetyl-D-mannosamine undergoes a NAD dependent dehydrogenation through a UDP-N-acetyl-D-mannosamine dehydrogenase, releasing NADH, a hydrogen ion and a UDP-N-Acetyl-alpha-D-mannosaminuronate, This compound is then used in the production of enterobacterial common antigens.
UDP-N-Acetyl-alpha-D-glucosamine-enolpyruvate is reduced through a NADPH dependent UDP-N-acetylenolpyruvoylglucosamine reductase, releasing a NADP and a UDP-N-acetyl-alpha-D-muramate. This compound is involved in the D-glutamine and D-glutamate metabolism.
|
Metabolite
Metabolic
|
|
|
SMP0440577![]() |
Sulfur MetabolismChlamydia trachomatis 434/Bu
The sulfur metabolism pathway starts in three possible ways. The first is the uptake of sulfate through an active transport reaction via a sulfate transport system containing an ATP-binding protein which hydrolyses ATP. Sulfate is converted by the sulfate adenylyltransferase enzymatic complex to adenosine phosphosulfate through the addition of adenine from a molecule of ATP, along with one phosphate group. Adenosine phosphosulfate is further converted to phoaphoadenosine phosphosulfate through an ATP hydrolysis and dehydrogenation reaction by the adenylyl-sulfate kinase. Phoaphoadenosine phosphosulfate is finally dehydrogenated and converted to sulfite by phosphoadenosine phosphosulfate reductase. This reaction requires magnesium, and adenosine 3',5'-diphosphate is the bi-product. A thioredoxin is also oxidized. Sulfite can also be produced from the dehydrogenation of cyanide along with the conversion of thiosulfate to thiocyanate by the thiosulfate sulfurtransferase enzymatic complex. Sulfite next undergoes a series of reactions that lead to the production of pyruvic acid, which is a precursor for pathways such as gluconeogenesis. The first reaction in this series is the conversion of sulfite to hydrogen sulfide through hygrogenation and the deoxygenation of sulfite to form a water molecule. The reaction is catalyzed by the sulfite reductase [NADPH] flavoprotein alpha and beta components. Siroheme, 4Fe-4S, flavin mononucleotide, and FAD function as cofactors or prosthetic groups. Hydrogen sulfide next undergoes dehydrogenation in a reversible reaction to form L-Cysteine and acetic acid, via the cysteine synthase complex and the coenzyme pyridoxal 5'-phosphate. L-Cysteine is dehydrogenated and converted to 2-aminoacrylic acid (a bronsted acid) and hydrogen sulfide(which may be reused) by a larger enzymatic complex composed of cysteine synthase A/B, protein malY, cystathionine-β-lyase, and tryptophanase, along with the coenzyme pyridoxal 5'-phosphate. 2-aminoacrylic acid isomerizes to 2-iminopropanoate, which along with a water molecule and a hydrogen ion is lastly converted to pyruvic acid and ammonium in a spontaneous fashion.
The second possible initial starting point for sulfur metabolism is the import of taurine(an alternate sulfur source) into the cytoplasm via the taurine ABC transporter complex. Taurine, oxoglutaric acid, and oxygen are converted to sulfite by the alpha-ketoglutarate-dependent taurine dioxygenase. Carbon dioxide, succinic acid, and aminoacetaldehyde are bi-products of this reaction. Sulfite next enters pyruvic acid synthesis as already described.
The third variant of sulfur metabolism starts with the import of an alkyl sulfate into the cytoplasm via an aliphatic sulfonate ABC transporter complex which hydrolyses ATP. The alkyl sulfate is dehydrogenated and along with oxygen is converted to sulfite and an aldehyde by the FMNH2-dependent alkanesulfonate monooxygenase enzyme. Water and flavin mononucleotide(which is used in a subsequent reaction as a prosthetic group) are also produced. Sulfite is next converted to pyruvic acid by the process already described.
|
Metabolite
Metabolic
|
|
|
SMP0437219![]() |
Hexuronide and Hexuronate DegradationBacteroides sp. 2_2_4
Beta-D-glucuronosides, D-glucuronate and D-fructuronate can be used as a source of carbon for E.coli. They are imported into E.coli's periplasmic space by membrane-associated protein (UidC/gusC), and are further imported into cytoplasm by hydrogen symporter. Beta-glucuronides undergoes hydrolysis by beta-D-glucuronidase to form D-glucuronate. D-glucuronate is isomerized by D-glucuronate isomerase to form D-fructuronate. D-fructuronate is further reduced to D-mannonate by D-mannonate oxidoreductase. D-mannonate dehydratase dehydrated to yield 2-dehydro-3-deoxy-D-gluconate. At this point, a common enzyme, 2-keto-3-deoxygluconokinase, phosphorylates 2-dehydro-3-deoxy-D-gluconate to yield 2-dehydro-3-deoxy-D-gluconate-6-phosphate. This product is then process by KHG/KDPG aldolase which in turn produces D-Glyceraldehyde 3-phosphate and Pyruvic Acid which then go into their respective sub pathways: glycolysis and pyruvate dehydrogenase. The pathway can also start from 3 other points: a hydrogen ion symporter (gluconate/fructuronate transporter GntP) of D-fructuronate, a hydrogen ion symporter (Hexuronate transporter) of aldehydo-D-galacturonate that spontaneously turns into D-tagaturonate. This compound can also be obtained by the reaction of aldehydo-L-galactonate with a NAD dependent l-galactonate oxidoreductase resulting in the release of NADH, hydrogen ion. Tagaturonate then undergoes an NADH-dependent reduction to D-altronate through an altronate oxidoreductase. D-altronate undergoes dehydration to yield 2-dehydro-3-deoxy-D-gluconate, the third and last point where the reaction can start from a hydrogen symporter of a 2-dehydro-3-deoy-D-gluconate.
|
Metabolite
Metabolic
|
|
|
SMP0437221![]() |
Hexuronide and Hexuronate DegradationBacteroides sp. 1_1_30
Beta-D-glucuronosides, D-glucuronate and D-fructuronate can be used as a source of carbon for E.coli. They are imported into E.coli's periplasmic space by membrane-associated protein (UidC/gusC), and are further imported into cytoplasm by hydrogen symporter. Beta-glucuronides undergoes hydrolysis by beta-D-glucuronidase to form D-glucuronate. D-glucuronate is isomerized by D-glucuronate isomerase to form D-fructuronate. D-fructuronate is further reduced to D-mannonate by D-mannonate oxidoreductase. D-mannonate dehydratase dehydrated to yield 2-dehydro-3-deoxy-D-gluconate. At this point, a common enzyme, 2-keto-3-deoxygluconokinase, phosphorylates 2-dehydro-3-deoxy-D-gluconate to yield 2-dehydro-3-deoxy-D-gluconate-6-phosphate. This product is then process by KHG/KDPG aldolase which in turn produces D-Glyceraldehyde 3-phosphate and Pyruvic Acid which then go into their respective sub pathways: glycolysis and pyruvate dehydrogenase. The pathway can also start from 3 other points: a hydrogen ion symporter (gluconate/fructuronate transporter GntP) of D-fructuronate, a hydrogen ion symporter (Hexuronate transporter) of aldehydo-D-galacturonate that spontaneously turns into D-tagaturonate. This compound can also be obtained by the reaction of aldehydo-L-galactonate with a NAD dependent l-galactonate oxidoreductase resulting in the release of NADH, hydrogen ion. Tagaturonate then undergoes an NADH-dependent reduction to D-altronate through an altronate oxidoreductase. D-altronate undergoes dehydration to yield 2-dehydro-3-deoxy-D-gluconate, the third and last point where the reaction can start from a hydrogen symporter of a 2-dehydro-3-deoy-D-gluconate.
|
Metabolite
Metabolic
|
|
|
SMP0437232![]() |
Amino Sugar and Nucleotide Sugar Metabolism IBacteroides sp. 2_2_4
The synthesis of amino sugars and nucleotide sugars starts with the phosphorylation of N-Acetylmuramic acid (MurNac) through its transport from the periplasmic space to the cytoplasm. Once in the cytoplasm, MurNac and water undergo a reversible reaction through a N-acetylmuramic acid 6-phosphate etherase, producing a D-lactic acid and N-Acetyl-D-Glucosamine 6-phosphate. This latter compound can also be introduced into the cytoplasm through a phosphorylating PTS permase in the inner membrane that allows for the transport of N-Acetyl-D-glucosamine from the periplasmic space. N-Acetyl-D-Glucosamine 6-phosphate can also be obtained from chitin dependent reactions. Chitin is hydrated through a bifunctional chitinase to produce chitobiose. This in turn gets hydrated by a beta-hexosaminidase to produce N-acetyl-D-glucosamine. The latter undergoes an atp dependent phosphorylation leading to the production of N-Acetyl-D-Glucosamine 6-phosphate.
N-Acetyl-D-Glucosamine 6-phosphate is then be deacetylated in order to produce Glucosamine 6-phosphate through a N-acetylglucosamine-6-phosphate deacetylase. This compound can either be isomerized or deaminated into Beta-D-fructofuranose 6-phosphate through a glucosamine-fructose-6-phosphate aminotransferase and a glucosamine-6-phosphate deaminase respectively.
Glucosamine 6-phosphate undergoes a reversible reaction to glucosamine 1 phosphate through a phosphoglucosamine mutase. This compound is then acetylated through a bifunctional protein glmU to produce a N-Acetyl glucosamine 1-phosphate.
N-Acetyl glucosamine 1-phosphate enters the nucleotide sugar synthesis by reacting with UTP and hydrogen ion through a bifunctional protein glmU releasing pyrophosphate and a Uridine diphosphate-N-acetylglucosamine.This compound can either be isomerized into a UDP-N-acetyl-D-mannosamine or undergo a reaction with phosphoenolpyruvic acid through UDP-N-acetylglucosamine 1-carboxyvinyltransferase releasing a phosphate and a UDP-N-Acetyl-alpha-D-glucosamine-enolpyruvate.
UDP-N-acetyl-D-mannosamine undergoes a NAD dependent dehydrogenation through a UDP-N-acetyl-D-mannosamine dehydrogenase, releasing NADH, a hydrogen ion and a UDP-N-Acetyl-alpha-D-mannosaminuronate, This compound is then used in the production of enterobacterial common antigens.
UDP-N-Acetyl-alpha-D-glucosamine-enolpyruvate is reduced through a NADPH dependent UDP-N-acetylenolpyruvoylglucosamine reductase, releasing a NADP and a UDP-N-acetyl-alpha-D-muramate. The latter is also involved in the D-glutamine and D-glutamate metabolism.
|
Metabolite
Metabolic
|
|
|
SMP0437239![]() |
Amino Sugar and Nucleotide Sugar Metabolism IIIBacteroides sp. 1_1_30
The synthesis of amino sugars and nucleotide sugars starts with the phosphorylation of N-Acetylmuramic acid (MurNac) through its transport from the periplasmic space to the cytoplasm. Once in the cytoplasm, MurNac and water undergo a reversible reaction catalyzed by N-acetylmuramic acid 6-phosphate etherase, producing a D-lactic acid and N-Acetyl-D-Glucosamine 6-phosphate. This latter compound can also be introduced into the cytoplasm through a phosphorylating PTS permase in the inner membrane that allows for the transport of N-Acetyl-D-glucosamine from the periplasmic space. N-Acetyl-D-Glucosamine 6-phosphate can also be obtained from chitin dependent reactions. Chitin is hydrated through a bifunctional chitinase to produce chitobiose. This in turn gets hydrated by a beta-hexosaminidase to produce N-acetyl-D-glucosamine. The latter undergoes an atp dependent phosphorylation leading to the production of N-Acetyl-D-Glucosamine 6-phosphate. N-Acetyl-D-Glucosamine 6-phosphate is then be deacetylated in order to produce Glucosamine 6-phosphate through a N-acetylglucosamine-6-phosphate deacetylase. This compound can either be isomerized or deaminated into Beta-D-fructofuranose 6-phosphate through a glucosamine-fructose-6-phosphate aminotransferase and a glucosamine-6-phosphate deaminase respectively.
Glucosamine 6-phosphate undergoes a reversible reaction to glucosamine 1 phosphate through a phosphoglucosamine mutase. This compound is then acetylated through a bifunctional protein glmU to produce a N-Acetyl glucosamine 1-phosphate.
N-Acetyl glucosamine 1-phosphate enters the nucleotide sugar synthesis by reacting with UTP and hydrogen ion through a bifunctional protein glmU releasing pyrophosphate and a Uridine diphosphate-N-acetylglucosamine.This compound can either be isomerized into a UDP-N-acetyl-D-mannosamine or undergo a reaction with phosphoenolpyruvic acid through UDP-N-acetylglucosamine 1-carboxyvinyltransferase releasing a phosphate and a UDP-N-Acetyl-alpha-D-glucosamine-enolpyruvate.
UDP-N-acetyl-D-mannosamine undergoes a NAD dependent dehydrogenation through a UDP-N-acetyl-D-mannosamine dehydrogenase, releasing NADH, a hydrogen ion and a UDP-N-Acetyl-alpha-D-mannosaminuronate, This compound is then used in the production of enterobacterial common antigens.
UDP-N-Acetyl-alpha-D-glucosamine-enolpyruvate is reduced through a NADPH dependent UDP-N-acetylenolpyruvoylglucosamine reductase, releasing a NADP and a UDP-N-acetyl-alpha-D-muramate. This compound is involved in the D-glutamine and D-glutamate metabolism.
|
Metabolite
Metabolic
|
|
|
SMP0437237![]() |
Amino Sugar and Nucleotide Sugar Metabolism IIBacteroides sp. 2_1_22
The synthesis of amino sugars and nucleotide sugars starts with the phosphorylation of N-Acetylmuramic acid (MurNac) through its transport from the periplasmic space to the cytoplasm. Once in the cytoplasm, MurNac and water undergo a reversible reaction through a N-acetylmuramic acid 6-phosphate etherase, producing a D-lactic acid and N-Acetyl-D-Glucosamine 6-phosphate. This latter compound can also be introduced into the cytoplasm through a phosphorylating PTS permase in the inner membrane that allows for the transport of N-Acetyl-D-glucosamine from the periplasmic space. N-Acetyl-D-Glucosamine 6-phosphate can also be obtained from chitin dependent reactions. Chitin is hydrated through a bifunctional chitinase to produce chitobiose. This in turn gets hydrated by a beta-hexosaminidase to produce N-acetyl-D-glucosamine. The latter undergoes an atp dependent phosphorylation leading to the production of N-Acetyl-D-Glucosamine 6-phosphate.
N-Acetyl-D-Glucosamine 6-phosphate is then be deacetylated in order to produce Glucosamine 6-phosphate through a N-acetylglucosamine-6-phosphate deacetylase. This compound can either be isomerized or deaminated into Beta-D-fructofuranose 6-phosphate through a glucosamine-fructose-6-phosphate aminotransferase and a glucosamine-6-phosphate deaminase respectively.
Glucosamine 6-phosphate undergoes a reversible reaction to glucosamine 1 phosphate through a phosphoglucosamine mutase. This compound is then acetylated through a bifunctional protein glmU to produce a N-Acetyl glucosamine 1-phosphate.
N-Acetyl glucosamine 1-phosphate enters the nucleotide sugar synthesis by reacting with UTP and hydrogen ion through a bifunctional protein glmU releasing pyrophosphate and a Uridine diphosphate-N-acetylglucosamine.This compound can either be isomerized into a UDP-N-acetyl-D-mannosamine or undergo a reaction with phosphoenolpyruvic acid through UDP-N-acetylglucosamine 1-carboxyvinyltransferase releasing a phosphate and a UDP-N-Acetyl-alpha-D-glucosamine-enolpyruvate.
UDP-N-acetyl-D-mannosamine undergoes a NAD dependent dehydrogenation through a UDP-N-acetyl-D-mannosamine dehydrogenase, releasing NADH, a hydrogen ion and a UDP-N-Acetyl-alpha-D-mannosaminuronate, This compound is then used in the production of enterobacterial common antigens.
UDP-N-Acetyl-alpha-D-glucosamine-enolpyruvate is reduced through a NADPH dependent UDP-N-acetylenolpyruvoylglucosamine reductase, releasing a NADP and a UDP-N-acetyl-alpha-D-muramate. This compound is involved in the D-glutamine and D-glutamate metabolism.
|
Metabolite
Metabolic
|
|
|
SMP0437226![]() |
S-Adenosyl-L-Methionine BiosynthesisBacteroides sp. 2_1_22
S-adenosyl-L-methionine biosynthesis(SAM) is synthesized in the cytosol of the cell from L-methionine and ATP. This reaction is catalyzed by methionine adenosyltransferase. L methione is taken up from the environment through a complex reaction coupled transport and then proceeds too synthesize the s adenosylmethionine through a adenosylmethionine synthase. S-adenosylmethionine then interacts with a hydrogen ion through an adenosylmethionine decarboxylase resulting in a carbon dioxide and a S-adenosyl 3-methioninamine. This compound interacts with a putrescine through a spermidine synthase resulting in a spermidine, a hydrogen ion and a S-methyl-5'-thioadenosine. The latter compound is degraded by interacting with a water molecule through a 5' methylthioadenosine nucleosidase resulting in an adenine and a S-methylthioribose which is then release into the environment
|
Metabolite
Metabolic
|
|
|
SMP0437217![]() |
PRPP BiosynthesisBacteroides xylanisolvens SD CC 1b
The biosynthesis of phosphoribosyl pyrophosphate begins as a product of the pentose phosphate and D-ribose 5-phosphate interaction. When catalyzed with a phosphopentomutase, the product is a ribose 1-phosphate. Ribose 1-phosphate can interact spontaneously with ATP resulting in a release of hydrogen ion, ADP and a ribose 1,5-biphosphate. Ribose 1,5-biphosphate is then phosphorylated through a ribose 1,5-bisphosphokinase resulting in the release of ADP and phosphoribosyl pyrophosphate. Phosphoribosyl pyrophosphate will then participate in the purine nucleotides de novo biosynthesis pathway. Alternatively pentose phosphate and D-ribose 5-phosphate's interaction can be phosphorylated through an ATP driven ribose-phosphate diphosphokinase resulting in a release of a hydrogen ion, an AMP and a phosphoribosyl pyrophosphate which will again participate in the purine nucleotides de novo biosynthesis pathway.
|
Metabolite
Metabolic
|
||
SMP0437384![]() |
Glutathione MetabolismPorphyromonas uenonis DSM 23387 = JCM 13868
The biosynthesis of glutathione starts with the introduction of L-glutamic acid through either a glutamate:sodium symporter, glutamate / aspartate : H+ symporter GltP or a
glutamate / aspartate ABC transporter. Once in the cytoplasm, L-glutamice acid reacts with L-cysteine through an ATP glutamate-cysteine ligase resulting in gamma-glutamylcysteine. This compound reacts which Glycine through an ATP driven glutathione synthetase thus catabolizing Glutathione.
This compound is metabolized through a spontaneous reaction with an oxidized glutaredoxin resulting in a reduced glutaredoxin and an oxidized glutathione. This compound is reduced by a NADPH glutathione reductase resulting in a glutathione.
Glutathione can then be degraded into various different glutathione containing compounds by reacting with a napthalene or Bromobenzene-2,3-oxide through a glutathione S-transferase.
|
Metabolite
Metabolic
|
|
Showing 367641 -
367650 of 369109 pathways