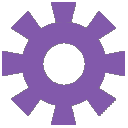
Browsing Pathways
Showing 368191 -
368200 of 605359 pathways
PathBank ID | Pathway Name and Description | Pathway Class | Chemical Compounds | Proteins |
---|---|---|---|---|
SMP0435638![]() |
Glutathione MetabolismAcinetobacter baumannii AYE
The biosynthesis of glutathione starts with the introduction of L-glutamic acid through either a glutamate:sodium symporter, glutamate / aspartate : H+ symporter GltP or a
glutamate / aspartate ABC transporter. Once in the cytoplasm, L-glutamice acid reacts with L-cysteine through an ATP glutamate-cysteine ligase resulting in gamma-glutamylcysteine. This compound reacts which Glycine through an ATP driven glutathione synthetase thus catabolizing Glutathione.
This compound is metabolized through a spontaneous reaction with an oxidized glutaredoxin resulting in a reduced glutaredoxin and an oxidized glutathione. This compound is reduced by a NADPH glutathione reductase resulting in a glutathione.
Glutathione can then be degraded into various different glutathione containing compounds by reacting with a napthalene or Bromobenzene-2,3-oxide through a glutathione S-transferase.
|
Metabolite
Metabolic
|
|
|
SMP0435609![]() |
Fatty Acid BiosynthesisBacteroides sp. 2_1_33B
The fatty acid biosynthesis starts from acetyl-CoA reacting either with a holo-[acp] through a 3-oxoacyl-[acp] synthase 3 resulting in an acetyl-[acp] or react with hydrogen carbonate through an ATP driven acetyl-CoA carboxylase resulting in a malonyl-CoA.
Malonyl-CoA reacts with a holo-acp] through a malonyl-CoA-ACP transacylase resulting in a malonyl-[acp]. This compound can react with a KASI protein resulting in an acetyl-[acp]. A malonyl-[acp] can also react with an acetyl-[acp] through KASI and KASII or with acetyl-CoA through a beta-ketoacyl-ACP synthase to produce an acetoacetyl-[acp]. An acetoacetyl-[acp] is also known as a 3-oxoacyl-[acp].
A 3-oxoacyl-[acp] is reduced through a NDPH mediated 3-oxoacyl-[acp] reductase resulting in a (3R)-3-hydroxyacyl-[acp] (R3 hydroxydecanoyl-[acp]) which can either join the fatty acid metabolism, be dehydrated by an 3R-hydroxymyristoyl-[acp] dehydratase to produce a trans-2-enoyl-[acp] or be dehydrated by a hydroxydecanoyl-[acp] to produce a trans-delta2 decenoyl-[acp].
Trans-2-enoyl-[acp] is reduced by a NADH driven enoyl-[acp] reductase resulting in a 2,3,4-saturated fatty acyl-[acp]. This product then reacts with malonyl-[acp] through KASI and KASII resulting in a holo-acyl carrier protein and a 3- oxoacyl-[acp].
Trans-delta2 decenoyl-[acp] reacts with a 3-hydroxydecanoyl-[acp] dehydrase producing a cis-delta 3-decenoyl-ACP. This product then reacts with KASI to produce a 3-oxo-cis-delta5-dodecenoyl-[acp], which in turn is reduced by a NADPH driven 3-oxoacyl-[acp] resulting in a 3R-hydroxy cis delta5-dodecenoyl-acp. This product is dehydrated by a (3R)-hydroxymyristoyl-[acp] dehydratase resulting in a trans-delta 3- cis-delta 5-dodecenoyl-[acp] which in turn is reduced by a NADH driven enoyl-[acp] reductase resulting in a cis-delta5-dodecenoyl-acp which becomes a metabolite of fatty acid metabolism
|
Metabolite
Metabolic
|
||
SMP0435616![]() |
Amino Sugar and Nucleotide Sugar Metabolism IIBacteroides intestinalis
The synthesis of amino sugars and nucleotide sugars starts with the phosphorylation of N-Acetylmuramic acid (MurNac) through its transport from the periplasmic space to the cytoplasm. Once in the cytoplasm, MurNac and water undergo a reversible reaction through a N-acetylmuramic acid 6-phosphate etherase, producing a D-lactic acid and N-Acetyl-D-Glucosamine 6-phosphate. This latter compound can also be introduced into the cytoplasm through a phosphorylating PTS permase in the inner membrane that allows for the transport of N-Acetyl-D-glucosamine from the periplasmic space. N-Acetyl-D-Glucosamine 6-phosphate can also be obtained from chitin dependent reactions. Chitin is hydrated through a bifunctional chitinase to produce chitobiose. This in turn gets hydrated by a beta-hexosaminidase to produce N-acetyl-D-glucosamine. The latter undergoes an atp dependent phosphorylation leading to the production of N-Acetyl-D-Glucosamine 6-phosphate.
N-Acetyl-D-Glucosamine 6-phosphate is then be deacetylated in order to produce Glucosamine 6-phosphate through a N-acetylglucosamine-6-phosphate deacetylase. This compound can either be isomerized or deaminated into Beta-D-fructofuranose 6-phosphate through a glucosamine-fructose-6-phosphate aminotransferase and a glucosamine-6-phosphate deaminase respectively.
Glucosamine 6-phosphate undergoes a reversible reaction to glucosamine 1 phosphate through a phosphoglucosamine mutase. This compound is then acetylated through a bifunctional protein glmU to produce a N-Acetyl glucosamine 1-phosphate.
N-Acetyl glucosamine 1-phosphate enters the nucleotide sugar synthesis by reacting with UTP and hydrogen ion through a bifunctional protein glmU releasing pyrophosphate and a Uridine diphosphate-N-acetylglucosamine.This compound can either be isomerized into a UDP-N-acetyl-D-mannosamine or undergo a reaction with phosphoenolpyruvic acid through UDP-N-acetylglucosamine 1-carboxyvinyltransferase releasing a phosphate and a UDP-N-Acetyl-alpha-D-glucosamine-enolpyruvate.
UDP-N-acetyl-D-mannosamine undergoes a NAD dependent dehydrogenation through a UDP-N-acetyl-D-mannosamine dehydrogenase, releasing NADH, a hydrogen ion and a UDP-N-Acetyl-alpha-D-mannosaminuronate, This compound is then used in the production of enterobacterial common antigens.
UDP-N-Acetyl-alpha-D-glucosamine-enolpyruvate is reduced through a NADPH dependent UDP-N-acetylenolpyruvoylglucosamine reductase, releasing a NADP and a UDP-N-acetyl-alpha-D-muramate. This compound is involved in the D-glutamine and D-glutamate metabolism.
|
Metabolite
Metabolic
|
|
|
SMP0435641![]() |
Hexuronide and Hexuronate DegradationAcinetobacter baumannii SDF
Beta-D-glucuronosides, D-glucuronate and D-fructuronate can be used as a source of carbon for E.coli. They are imported into E.coli's periplasmic space by membrane-associated protein (UidC/gusC), and are further imported into cytoplasm by hydrogen symporter. Beta-glucuronides undergoes hydrolysis by beta-D-glucuronidase to form D-glucuronate. D-glucuronate is isomerized by D-glucuronate isomerase to form D-fructuronate. D-fructuronate is further reduced to D-mannonate by D-mannonate oxidoreductase. D-mannonate dehydratase dehydrated to yield 2-dehydro-3-deoxy-D-gluconate. At this point, a common enzyme, 2-keto-3-deoxygluconokinase, phosphorylates 2-dehydro-3-deoxy-D-gluconate to yield 2-dehydro-3-deoxy-D-gluconate-6-phosphate. This product is then process by KHG/KDPG aldolase which in turn produces D-Glyceraldehyde 3-phosphate and Pyruvic Acid which then go into their respective sub pathways: glycolysis and pyruvate dehydrogenase. The pathway can also start from 3 other points: a hydrogen ion symporter (gluconate/fructuronate transporter GntP) of D-fructuronate, a hydrogen ion symporter (Hexuronate transporter) of aldehydo-D-galacturonate that spontaneously turns into D-tagaturonate. This compound can also be obtained by the reaction of aldehydo-L-galactonate with a NAD dependent l-galactonate oxidoreductase resulting in the release of NADH, hydrogen ion. Tagaturonate then undergoes an NADH-dependent reduction to D-altronate through an altronate oxidoreductase. D-altronate undergoes dehydration to yield 2-dehydro-3-deoxy-D-gluconate, the third and last point where the reaction can start from a hydrogen symporter of a 2-dehydro-3-deoy-D-gluconate.
|
Metabolite
Metabolic
|
|
|
SMP0435633![]() |
PRPP BiosynthesisAchromobacter xylosoxidans A8
The biosynthesis of phosphoribosyl pyrophosphate begins as a product of the pentose phosphate and D-ribose 5-phosphate interaction. When catalyzed with a phosphopentomutase, the product is a ribose 1-phosphate. Ribose 1-phosphate can interact spontaneously with ATP resulting in a release of hydrogen ion, ADP and a ribose 1,5-biphosphate. Ribose 1,5-biphosphate is then phosphorylated through a ribose 1,5-bisphosphokinase resulting in the release of ADP and phosphoribosyl pyrophosphate. Phosphoribosyl pyrophosphate will then participate in the purine nucleotides de novo biosynthesis pathway. Alternatively pentose phosphate and D-ribose 5-phosphate's interaction can be phosphorylated through an ATP driven ribose-phosphate diphosphokinase resulting in a release of a hydrogen ion, an AMP and a phosphoribosyl pyrophosphate which will again participate in the purine nucleotides de novo biosynthesis pathway.
|
Metabolite
Metabolic
|
||
SMP0436369![]() |
Purine Nucleotides De Novo BiosynthesisEscherichia coli str. K-12 substr. MG1655
The biosynthesis of purine nucleotides is a complex process that begins with a phosphoribosyl pyrophosphate. This compound interacts with water and L-glutamine through a
amidophosphoribosyl transferase resulting in a pyrophosphate, L-glutamic acid and a 5-phosphoribosylamine. The latter compound proceeds to interact with a glycine through an ATP driven phosphoribosylamine-glycine ligase resulting in the addition of glycine to the compound. This reaction releases an ADP, a phosphate, a hydrogen ion and a N1-(5-phospho-β-D-ribosyl)glycinamide. The latter compound interacts with formic acid, through an ATP driven phosphoribosylglycinamide formyltransferase 2 resulting in a phosphate, an ADP, a hydrogen ion and a 5-phosphoribosyl-N-formylglycinamide. The latter compound interacts with L-glutamine, and water through an ATP-driven
phosphoribosylformylglycinamide synthetase resulting in a release of a phosphate, an ADP, a hydrogen ion, a L-glutamic acid and a 2-(formamido)-N1-(5-phospho-D-ribosyl)acetamidine. The latter compound interacts with an ATP driven phosphoribosylformylglycinamide cyclo-ligase resulting in a release of ADP, a phosphate, a hydrogen ion and a 5-aminoimidazole ribonucleotide. The latter compound interacts with a hydrogen carbonate through an ATP driven N5-carboxyaminoimidazole ribonucleotide synthetase resulting in a release of a phosphate, an ADP, a hydrogen ion and a N5-carboxyaminoimidazole ribonucleotide.The latter compound then interacts with a N5-carboxyaminoimidazole ribonucleotide mutase resulting in a 5-amino-1-(5-phospho-D-ribosyl)imidazole-4-carboxylate. This compound interacts with an L-aspartic acid through an ATP driven phosphoribosylaminoimidazole-succinocarboxamide synthase resulting in a phosphate, an ADP, a hydrogen ion and a SAICAR. SAICAR interacts with an adenylosuccinate lyase resulting in a fumaric acid and an AICAR. AICAR interacts with a formyltetrahydrofolate through a AICAR transformylase / IMP cyclohydrolase resulting in a release of a tetrahydropterol mono-l-glutamate and a FAICAR. The latter compound, FAICAR, interacts in a reversible reaction through a AICAR transformylase / IMP cyclohydrolase resulting in a release of water and Inosinic acid.
Inosinic acid can be metabolized to produce dGTP and dATP three different methods each.
dGTP:
Inosinic acid, water and NAD are processed by IMP dehydrogenase resulting in a release of NADH, a hydrogen ion and Xanthylic acid. Xanthylic acid interacts with L-glutamine, and water through an ATP driven GMP synthetase resulting in pyrophosphate, AMP, L-glutamic acid, a hydrogen ion and Guanosine monophosphate. The latter compound is the phosphorylated by reacting with an ATP driven guanylate kinase resulting in a release of ADP and a Gaunosine diphosphate. Guanosine diphosphate can be metabolized in three different ways:
1.-Guanosine diphosphate is phosphorylated by an ATP-driven nucleoside diphosphate kinase resulting in an ADP and a Guanosine triphosphate. This compound interacts with a reduced flavodoxin protein through a ribonucleoside-triphosphate reductase resulting in a oxidized flavodoxin a water moleculer and a dGTP
2.-Guanosine diphosphate interacts with a reduced NrdH glutaredoxin-like proteins through a ribonucleoside-diphosphate reductase 2 resulting in the release of an oxidized NrdH glutaredoxin-like protein, a water molecule and a dGDP. The dGDP is then phosphorylated by interacting with an ATP-driven nucleoside diphosphate kinase resulting in an ADP and dGTP.
3.-Guanosine diphosphate interacts with a reduced thioredoxin ribonucleoside diphosphate reductase 1 resulting in a release of a water molecule, an oxidized thioredoxin and a dGDP. The dGDP is then phosphorylated by interacting with an ATP-driven nucleoside diphosphate kinase resulting in an ADP and dGTP.
dATP:
Inosinic acid interacts with L-aspartic acid through an GTP driven adenylosuccinate synthase results in the release of GDP, a hydrogen ion, a phosphate and N(6)-(1,2-dicarboxyethyl)AMP. The latter compound is then cleaved by a adenylosuccinate lyase resulting in a fumaric acid and an Adenosine monophosphate. This compound is then phosphorylated by an adenylate kinase resulting in the release of ATP and an adenosine diphosphate. Adenosine diphosphate can be metabolized in three different ways:
1.-Adenosine diphosphate is involved in a reversible reaction by interacting with a hydrogen ion and a phosphate through a ATP synthase / thiamin triphosphate synthase resulting in a hydrogen ion, a water molecule and an Adenosine triphosphate. The adenosine triphosphate interacts with a reduced flavodoxin through a ribonucleoside-triphosphate reductase resulting in an oxidized flavodoxin, a water molecule and a dATP
2.- Adenosine diphosphate interacts with an reduced thioredoxin through a ribonucleoside diphosphate reductase 1 resulting in a release of a water molecule, a oxidized thioredoxin and a dADP. The dADP is then phosphorylated by a nucleoside diphosphate kinase resulting in the release of ADP and a dATP
3.- Adenosine diphosphate interacts with an reduced NrdH glutaredoxin-like protein through a ribonucleoside diphosphate reductase 2 resulting in a release of a water molecule, a oxidized glutaredoxin-like protein and a dADP. The dADP is then phosphorylated by a nucleoside diphosphate kinase resulting in the release of ADP and a dATP
|
Metabolite
Metabolic
|
|
|
SMP0436373![]() |
Fructose MetabolismEscherichia coli str. K-12 substr. MG1655
Fructose metabolism begins with the transport of Beta-D-fructofuranose through a fructose PTS permease, resulting in a Beta-D-fructofuranose 1-phosphate. This compound is phosphorylated by an ATP driven 1-phosphofructokinase resulting in a fructose 1,6-biphosphate. This compound can either react with a fructose bisphosphate aldolase class 1 resulting in D-glyceraldehyde 3-phosphate and a dihydroxyacetone phosphate or through a fructose biphosphate aldolase class 2 resulting in a D-glyceraldehyde 3-phosphate. This compound can then either react in a reversible triosephosphate isomerase resulting in a dihydroxyacetone phosphate or react with a phosphate through a NAD dependent Glyceraldehyde 3-phosphate dehydrogenase resulting in a glyceric acid 1,3-biphosphate. This compound is desphosphorylated by a phosphoglycerate kinase resulting in a 3-phosphoglyceric acid.This compound in turn can either react with a 2,3-bisphosphoglycerate-independent phosphoglycerate mutase or a 2,3-bisphosphoglycerate-independent phosphoglycerate mutase resulting in a 2-phospho-D-glyceric acid. This compound interacts with an enolase resulting in a phosphoenolpyruvic acid and water. Phosphoenolpyruvic acid can react either through a AMP driven phosphoenoylpyruvate synthase or a ADP driven pyruvate kinase protein complex resulting in a pyruvic acid.
Pyruvic acid reacts with CoA through a NAD driven pyruvate dehydrogenase complex resulting in a carbon dioxide and a Acetyl-CoA which gets incorporated into the TCA cycle pathway.
|
Metabolite
Metabolic
|
|
|
SMP0435912![]() |
S-Adenosyl-L-Methionine BiosynthesisCampylobacter jejuni subsp. jejuni 81-176
S-adenosyl-L-methionine biosynthesis(SAM) is synthesized in the cytosol of the cell from L-methionine and ATP. This reaction is catalyzed by methionine adenosyltransferase. L methione is taken up from the environment through a complex reaction coupled transport and then proceeds too synthesize the s adenosylmethionine through a adenosylmethionine synthase. S-adenosylmethionine then interacts with a hydrogen ion through an adenosylmethionine decarboxylase resulting in a carbon dioxide and a S-adenosyl 3-methioninamine. This compound interacts with a putrescine through a spermidine synthase resulting in a spermidine, a hydrogen ion and a S-methyl-5'-thioadenosine. The latter compound is degraded by interacting with a water molecule through a 5' methylthioadenosine nucleosidase resulting in an adenine and a S-methylthioribose which is then release into the environment
|
Metabolite
Metabolic
|
|
|
SMP0435915![]() |
Galactose Degradation/Leloir PathwayCampylobacter jejuni subsp. jejuni 81-176
The degradation of galactose, also known as Leloir pathway, requires 3 main enzymes once Beta-D-galactose has been converted to galactose through an Aldose-1-epimerase. These are: galactokinase , galactose-1-phosphate uridylyltransferase and UDP-glucose 4-epimerase. Beta-D-galactose can be uptaken from the environment through a galactose proton symporter. It can also be produced by lactose degradation involving a lactose permease to uptake lactose from the environment and a beta-galactosidase to turn lactose into Beta-D-galactose.
Galactose is degraded through the following process:
Beta-D-galactose is introduced into the cytoplasm through a galactose proton symporter, or it can be synthesized from an alpha lactose that is introduced into the cytoplasm through a lactose permease. Alpha lactose interacts with water through a beta-galactosidase resulting in a beta-D-glucose and beta-D-galactose. Beta-D-galactose is isomerized into D-galactose. D-Galactose undergoes phosphorylation through a galactokinase, hence producing galactose 1 phosphate. On the other side of the pathway, a gluose-1-phosphate (product of the interaction of alpha-D-glucose 6-phosphate with a phosphoglucomutase resulting in a alpha-D-glucose-1-phosphate, an isomer of Glucose 1-phosphate, or an isomer of Beta-D-glucose 1-phosphate) interacts with UTP and a hydrogen ion in order to produce a uridine diphosphate glucose. This is followed by the interaction of galactose-1-phosphate with an established amount of uridine diphosphate glucose through a galactose-1-phosphate uridylyltransferase, which in turn output a glucose-1-phosphate and a uridine diphosphate galactose. The glucose -1-phosphate is transformed into a uridine diphosphate glucose through UTP--glucose-1-phosphate uridylyltransferase. The product, uridine diphosphate glucose, can undergo a reversible reaction in which it can be turned into uridine diphosphategalactose through an UDP-glucose 4-epimerase, and so the cycle can keep going as long as more lactose or galactose is imported into the cell.
|
Metabolite
Metabolic
|
|
|
SMP0435904![]() |
Glutathione MetabolismCampylobacter jejuni subsp. jejuni 81-176
The biosynthesis of glutathione starts with the introduction of L-glutamic acid through either a glutamate:sodium symporter, glutamate / aspartate : H+ symporter GltP or a
glutamate / aspartate ABC transporter. Once in the cytoplasm, L-glutamice acid reacts with L-cysteine through an ATP glutamate-cysteine ligase resulting in gamma-glutamylcysteine. This compound reacts which Glycine through an ATP driven glutathione synthetase thus catabolizing Glutathione.
This compound is metabolized through a spontaneous reaction with an oxidized glutaredoxin resulting in a reduced glutaredoxin and an oxidized glutathione. This compound is reduced by a NADPH glutathione reductase resulting in a glutathione.
Glutathione can then be degraded into various different glutathione containing compounds by reacting with a napthalene or Bromobenzene-2,3-oxide through a glutathione S-transferase.
|
Metabolite
Metabolic
|
|
Showing 368191 -
368200 of 369097 pathways