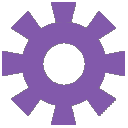
Browsing Pathways
Showing 368361 -
368370 of 605359 pathways
PathBank ID | Pathway Name and Description | Pathway Class | Chemical Compounds | Proteins |
---|---|---|---|---|
SMP0436116![]() |
Amino Sugar and Nucleotide Sugar Metabolism IChlamydia trachomatis 434/Bu
The synthesis of amino sugars and nucleotide sugars starts with the phosphorylation of N-Acetylmuramic acid (MurNac) through its transport from the periplasmic space to the cytoplasm. Once in the cytoplasm, MurNac and water undergo a reversible reaction through a N-acetylmuramic acid 6-phosphate etherase, producing a D-lactic acid and N-Acetyl-D-Glucosamine 6-phosphate. This latter compound can also be introduced into the cytoplasm through a phosphorylating PTS permase in the inner membrane that allows for the transport of N-Acetyl-D-glucosamine from the periplasmic space. N-Acetyl-D-Glucosamine 6-phosphate can also be obtained from chitin dependent reactions. Chitin is hydrated through a bifunctional chitinase to produce chitobiose. This in turn gets hydrated by a beta-hexosaminidase to produce N-acetyl-D-glucosamine. The latter undergoes an atp dependent phosphorylation leading to the production of N-Acetyl-D-Glucosamine 6-phosphate.
N-Acetyl-D-Glucosamine 6-phosphate is then be deacetylated in order to produce Glucosamine 6-phosphate through a N-acetylglucosamine-6-phosphate deacetylase. This compound can either be isomerized or deaminated into Beta-D-fructofuranose 6-phosphate through a glucosamine-fructose-6-phosphate aminotransferase and a glucosamine-6-phosphate deaminase respectively.
Glucosamine 6-phosphate undergoes a reversible reaction to glucosamine 1 phosphate through a phosphoglucosamine mutase. This compound is then acetylated through a bifunctional protein glmU to produce a N-Acetyl glucosamine 1-phosphate.
N-Acetyl glucosamine 1-phosphate enters the nucleotide sugar synthesis by reacting with UTP and hydrogen ion through a bifunctional protein glmU releasing pyrophosphate and a Uridine diphosphate-N-acetylglucosamine.This compound can either be isomerized into a UDP-N-acetyl-D-mannosamine or undergo a reaction with phosphoenolpyruvic acid through UDP-N-acetylglucosamine 1-carboxyvinyltransferase releasing a phosphate and a UDP-N-Acetyl-alpha-D-glucosamine-enolpyruvate.
UDP-N-acetyl-D-mannosamine undergoes a NAD dependent dehydrogenation through a UDP-N-acetyl-D-mannosamine dehydrogenase, releasing NADH, a hydrogen ion and a UDP-N-Acetyl-alpha-D-mannosaminuronate, This compound is then used in the production of enterobacterial common antigens.
UDP-N-Acetyl-alpha-D-glucosamine-enolpyruvate is reduced through a NADPH dependent UDP-N-acetylenolpyruvoylglucosamine reductase, releasing a NADP and a UDP-N-acetyl-alpha-D-muramate. The latter is also involved in the D-glutamine and D-glutamate metabolism.
|
Metabolite
Metabolic
|
|
|
SMP0436111![]() |
Galactose Degradation/Leloir PathwayCitrobacter koseri ATCC BAA-895
The degradation of galactose, also known as Leloir pathway, requires 3 main enzymes once Beta-D-galactose has been converted to galactose through an Aldose-1-epimerase. These are: galactokinase , galactose-1-phosphate uridylyltransferase and UDP-glucose 4-epimerase. Beta-D-galactose can be uptaken from the environment through a galactose proton symporter. It can also be produced by lactose degradation involving a lactose permease to uptake lactose from the environment and a beta-galactosidase to turn lactose into Beta-D-galactose.
Galactose is degraded through the following process:
Beta-D-galactose is introduced into the cytoplasm through a galactose proton symporter, or it can be synthesized from an alpha lactose that is introduced into the cytoplasm through a lactose permease. Alpha lactose interacts with water through a beta-galactosidase resulting in a beta-D-glucose and beta-D-galactose. Beta-D-galactose is isomerized into D-galactose. D-Galactose undergoes phosphorylation through a galactokinase, hence producing galactose 1 phosphate. On the other side of the pathway, a gluose-1-phosphate (product of the interaction of alpha-D-glucose 6-phosphate with a phosphoglucomutase resulting in a alpha-D-glucose-1-phosphate, an isomer of Glucose 1-phosphate, or an isomer of Beta-D-glucose 1-phosphate) interacts with UTP and a hydrogen ion in order to produce a uridine diphosphate glucose. This is followed by the interaction of galactose-1-phosphate with an established amount of uridine diphosphate glucose through a galactose-1-phosphate uridylyltransferase, which in turn output a glucose-1-phosphate and a uridine diphosphate galactose. The glucose -1-phosphate is transformed into a uridine diphosphate glucose through UTP--glucose-1-phosphate uridylyltransferase. The product, uridine diphosphate glucose, can undergo a reversible reaction in which it can be turned into uridine diphosphategalactose through an UDP-glucose 4-epimerase, and so the cycle can keep going as long as more lactose or galactose is imported into the cell.
|
Metabolite
Metabolic
|
|
|
SMP0436460![]() |
Oxidative PhosphorylationEscherichia coli K-12
The process of oxidative phosphorylation involves multiple interactions of ubiquinone with succinic acid, resulting in a fumaric acid and ubiquinol.
Ubiquinone interacts with succinic acid through a succinate:quinone oxidoreductase resulting in a fumaric acid an ubiquinol. This enzyme has various cofactors, ferroheme b, 2FE-2S, FAD, and 3Fe-4S iron-sulfur cluster.
Then 2 ubiquinol interact with oxygen and 4 hydrogen ion through a cytochrome bd-I terminal oxidase resulting in a 4 hydrogen ion transferred into the periplasmic space, 2 water returned into the cytoplasm and 2 ubiquinone, which stay in the inner membrane.
The ubiquinone interacts with succinic acid through a succinate:quinone oxidoreductase resulting in a fumaric acid an ubiquinol.
Then 2 ubiquinol interacts with oxygen and 4 hydrogen ion through a cytochrome bd-II terminal oxidase resulting in a 4 hydrogen ion transferred into the periplasmic space, 2 water returned into the cytoplasm and 2 ubiquinone, which stay in the inner membrane.
The ubiquinone interacts with succinic acid through a succinate:quinone oxidoreductase resulting in a fumaric acid an ubiquinol.
The 2 ubiquinol interact with oxygen and 8 hydrogen ion through a cytochrome bo terminal oxidase resulting in a 8 hydrogen ion transferred into the periplasmic space, 2 water returned into the cytoplasm and 2 ubiquinone, which stays in the inner membrane.
The ubiquinone then interacts with 5 hydrogen ion through a NADH dependent ubiquinone oxidoreductase I resulting in NAD, hydrogen ion released into the periplasmic space and an ubiquinol.
The ubiquinol is then processed reacting with oxygen, and 4 hydrogen through a ion cytochrome bd-I terminal oxidase resulting in 4 hydrogen ions released into the periplasmic space, 2 water molecules into the cytoplasm and 2 ubiquinones.
The ubiquinone then interacts with 5 hydrogen ion through a NADH dependent ubiquinone oxidoreductase I resulting in NAD, hydrogen ion released into the periplasmic space and an ubiquinol.
The 2 ubiquinol interact with oxygen and 8 hydrogen ion through a cytochrome bo terminal oxidase resulting in a 8 hydrogen ion transferred into the periplasmic space, 2 water returned into the cytoplasm and 2 ubiquinone, which stays in the inner membrane.
|
Metabolite
Metabolic
|
|
|
SMP0436102![]() |
Hexuronide and Hexuronate DegradationCitrobacter koseri ATCC BAA-895
Beta-D-glucuronosides, D-glucuronate and D-fructuronate can be used as a source of carbon for E.coli. They are imported into E.coli's periplasmic space by membrane-associated protein (UidC/gusC), and are further imported into cytoplasm by hydrogen symporter. Beta-glucuronides undergoes hydrolysis by beta-D-glucuronidase to form D-glucuronate. D-glucuronate is isomerized by D-glucuronate isomerase to form D-fructuronate. D-fructuronate is further reduced to D-mannonate by D-mannonate oxidoreductase. D-mannonate dehydratase dehydrated to yield 2-dehydro-3-deoxy-D-gluconate. At this point, a common enzyme, 2-keto-3-deoxygluconokinase, phosphorylates 2-dehydro-3-deoxy-D-gluconate to yield 2-dehydro-3-deoxy-D-gluconate-6-phosphate. This product is then process by KHG/KDPG aldolase which in turn produces D-Glyceraldehyde 3-phosphate and Pyruvic Acid which then go into their respective sub pathways: glycolysis and pyruvate dehydrogenase. The pathway can also start from 3 other points: a hydrogen ion symporter (gluconate/fructuronate transporter GntP) of D-fructuronate, a hydrogen ion symporter (Hexuronate transporter) of aldehydo-D-galacturonate that spontaneously turns into D-tagaturonate. This compound can also be obtained by the reaction of aldehydo-L-galactonate with a NAD dependent l-galactonate oxidoreductase resulting in the release of NADH, hydrogen ion. Tagaturonate then undergoes an NADH-dependent reduction to D-altronate through an altronate oxidoreductase. D-altronate undergoes dehydration to yield 2-dehydro-3-deoxy-D-gluconate, the third and last point where the reaction can start from a hydrogen symporter of a 2-dehydro-3-deoy-D-gluconate.
|
Metabolite
Metabolic
|
|
|
SMP0436122![]() |
Amino Sugar and Nucleotide Sugar Metabolism IICronobacter sakazakii ATCC BAA-894
The synthesis of amino sugars and nucleotide sugars starts with the phosphorylation of N-Acetylmuramic acid (MurNac) through its transport from the periplasmic space to the cytoplasm. Once in the cytoplasm, MurNac and water undergo a reversible reaction through a N-acetylmuramic acid 6-phosphate etherase, producing a D-lactic acid and N-Acetyl-D-Glucosamine 6-phosphate. This latter compound can also be introduced into the cytoplasm through a phosphorylating PTS permase in the inner membrane that allows for the transport of N-Acetyl-D-glucosamine from the periplasmic space. N-Acetyl-D-Glucosamine 6-phosphate can also be obtained from chitin dependent reactions. Chitin is hydrated through a bifunctional chitinase to produce chitobiose. This in turn gets hydrated by a beta-hexosaminidase to produce N-acetyl-D-glucosamine. The latter undergoes an atp dependent phosphorylation leading to the production of N-Acetyl-D-Glucosamine 6-phosphate.
N-Acetyl-D-Glucosamine 6-phosphate is then be deacetylated in order to produce Glucosamine 6-phosphate through a N-acetylglucosamine-6-phosphate deacetylase. This compound can either be isomerized or deaminated into Beta-D-fructofuranose 6-phosphate through a glucosamine-fructose-6-phosphate aminotransferase and a glucosamine-6-phosphate deaminase respectively.
Glucosamine 6-phosphate undergoes a reversible reaction to glucosamine 1 phosphate through a phosphoglucosamine mutase. This compound is then acetylated through a bifunctional protein glmU to produce a N-Acetyl glucosamine 1-phosphate.
N-Acetyl glucosamine 1-phosphate enters the nucleotide sugar synthesis by reacting with UTP and hydrogen ion through a bifunctional protein glmU releasing pyrophosphate and a Uridine diphosphate-N-acetylglucosamine.This compound can either be isomerized into a UDP-N-acetyl-D-mannosamine or undergo a reaction with phosphoenolpyruvic acid through UDP-N-acetylglucosamine 1-carboxyvinyltransferase releasing a phosphate and a UDP-N-Acetyl-alpha-D-glucosamine-enolpyruvate.
UDP-N-acetyl-D-mannosamine undergoes a NAD dependent dehydrogenation through a UDP-N-acetyl-D-mannosamine dehydrogenase, releasing NADH, a hydrogen ion and a UDP-N-Acetyl-alpha-D-mannosaminuronate, This compound is then used in the production of enterobacterial common antigens.
UDP-N-Acetyl-alpha-D-glucosamine-enolpyruvate is reduced through a NADPH dependent UDP-N-acetylenolpyruvoylglucosamine reductase, releasing a NADP and a UDP-N-acetyl-alpha-D-muramate. This compound is involved in the D-glutamine and D-glutamate metabolism.
|
Metabolite
Metabolic
|
|
|
SMP0436137![]() |
beta-Alanine MetabolismCitrobacter koseri ATCC BAA-895
Beta-Alanine metabolism starts as a product of aspartate metabolism. Aspartate is decarboxylated by aspartate 1-decarboxylase, releasing carbon dioxide and beta-alanine. Beta-Alanine is then metabolized through a pantothenate synthease resulting in pantothenic acid. Pantothenic acid then undergoes phosphorylation through an ATP-driven pantothenate kinase, resulting in D-4-phosphopantothenate. Pantothenate, vitamin B5, is a precursor for synthesis of 4'-phosphopantetheine moiety of coenzyme A and acyl carrier protein. Plants and microorganisms can synthesize pantothenate de novo, but animals must obtain it from diet. Enzymes of beta-alanine metabolism are targets for anti-microbial drugs.
|
Metabolite
Metabolic
|
||
SMP0436138![]() |
beta-Alanine MetabolismChlamydia trachomatis 434/Bu
Beta-Alanine metabolism starts as a product of aspartate metabolism. Aspartate is decarboxylated by aspartate 1-decarboxylase, releasing carbon dioxide and beta-alanine. Beta-Alanine is then metabolized through a pantothenate synthease resulting in pantothenic acid. Pantothenic acid then undergoes phosphorylation through an ATP-driven pantothenate kinase, resulting in D-4-phosphopantothenate. Pantothenate, vitamin B5, is a precursor for synthesis of 4'-phosphopantetheine moiety of coenzyme A and acyl carrier protein. Plants and microorganisms can synthesize pantothenate de novo, but animals must obtain it from diet. Enzymes of beta-alanine metabolism are targets for anti-microbial drugs.
|
Metabolite
Metabolic
|
||
SMP0436454![]() |
Galactose Degradation/Leloir PathwayEscherichia coli O26:H11 str. 11368
The degradation of galactose, also known as Leloir pathway, requires 3 main enzymes once Beta-D-galactose has been converted to galactose through an Aldose-1-epimerase. These are: galactokinase , galactose-1-phosphate uridylyltransferase and UDP-glucose 4-epimerase. Beta-D-galactose can be uptaken from the environment through a galactose proton symporter. It can also be produced by lactose degradation involving a lactose permease to uptake lactose from the environment and a beta-galactosidase to turn lactose into Beta-D-galactose.
Galactose is degraded through the following process:
Beta-D-galactose is introduced into the cytoplasm through a galactose proton symporter, or it can be synthesized from an alpha lactose that is introduced into the cytoplasm through a lactose permease. Alpha lactose interacts with water through a beta-galactosidase resulting in a beta-D-glucose and beta-D-galactose. Beta-D-galactose is isomerized into D-galactose. D-Galactose undergoes phosphorylation through a galactokinase, hence producing galactose 1 phosphate. On the other side of the pathway, a gluose-1-phosphate (product of the interaction of alpha-D-glucose 6-phosphate with a phosphoglucomutase resulting in a alpha-D-glucose-1-phosphate, an isomer of Glucose 1-phosphate, or an isomer of Beta-D-glucose 1-phosphate) interacts with UTP and a hydrogen ion in order to produce a uridine diphosphate glucose. This is followed by the interaction of galactose-1-phosphate with an established amount of uridine diphosphate glucose through a galactose-1-phosphate uridylyltransferase, which in turn output a glucose-1-phosphate and a uridine diphosphate galactose. The glucose -1-phosphate is transformed into a uridine diphosphate glucose through UTP--glucose-1-phosphate uridylyltransferase. The product, uridine diphosphate glucose, can undergo a reversible reaction in which it can be turned into uridine diphosphategalactose through an UDP-glucose 4-epimerase, and so the cycle can keep going as long as more lactose or galactose is imported into the cell.
|
Metabolite
Metabolic
|
|
|
SMP0436451![]() |
Galactose Degradation/Leloir PathwayEscherichia coli IAI39
The degradation of galactose, also known as Leloir pathway, requires 3 main enzymes once Beta-D-galactose has been converted to galactose through an Aldose-1-epimerase. These are: galactokinase , galactose-1-phosphate uridylyltransferase and UDP-glucose 4-epimerase. Beta-D-galactose can be uptaken from the environment through a galactose proton symporter. It can also be produced by lactose degradation involving a lactose permease to uptake lactose from the environment and a beta-galactosidase to turn lactose into Beta-D-galactose.
Galactose is degraded through the following process:
Beta-D-galactose is introduced into the cytoplasm through a galactose proton symporter, or it can be synthesized from an alpha lactose that is introduced into the cytoplasm through a lactose permease. Alpha lactose interacts with water through a beta-galactosidase resulting in a beta-D-glucose and beta-D-galactose. Beta-D-galactose is isomerized into D-galactose. D-Galactose undergoes phosphorylation through a galactokinase, hence producing galactose 1 phosphate. On the other side of the pathway, a gluose-1-phosphate (product of the interaction of alpha-D-glucose 6-phosphate with a phosphoglucomutase resulting in a alpha-D-glucose-1-phosphate, an isomer of Glucose 1-phosphate, or an isomer of Beta-D-glucose 1-phosphate) interacts with UTP and a hydrogen ion in order to produce a uridine diphosphate glucose. This is followed by the interaction of galactose-1-phosphate with an established amount of uridine diphosphate glucose through a galactose-1-phosphate uridylyltransferase, which in turn output a glucose-1-phosphate and a uridine diphosphate galactose. The glucose -1-phosphate is transformed into a uridine diphosphate glucose through UTP--glucose-1-phosphate uridylyltransferase. The product, uridine diphosphate glucose, can undergo a reversible reaction in which it can be turned into uridine diphosphategalactose through an UDP-glucose 4-epimerase, and so the cycle can keep going as long as more lactose or galactose is imported into the cell.
|
Metabolite
Metabolic
|
|
|
SMP0436419![]() |
Folate BiosynthesisEscherichia fergusonii ATCC 35469
The biosynthesis of folic acid begins as a product of purine nucleotides de novo biosynthesis pathway. Purine nucleotides are involved in a reaction with water through a GTP cyclohydrolase 1 protein complex, resulting in a hydrogen ion, formic acid and 7,8-dihydroneopterin 3-triphosphate. The latter compound is dephosphorylated through a dihydroneopterin triphosphate pyrophosphohydrolase resulting in the release of a pyrophosphate, hydrogen ion and 7,8-dihydroneopterin 3-phosphate. The latter product reacts with water spontaneously resulting in the release of a phosphate and a 7,8 -dihydroneopterin. 7,8 -dihydroneopterin reacts with a dihydroneopterin aldolase, releasing a glycoaldehyde and 6-hydroxymethyl-7,9-dihydropterin. Continuing, 6-hydroxymethyl-7,9-dihydropterin is phosphorylated with a ATP-driven 6-hydroxymethyl-7,8-dihydropterin pyrophosphokinase resulting in a (2-amino-4-hydroxy-7,8-dihydropteridin-6-yl)methyl diphosphate.
Chorismate is metabolized by reacting with L-glutamine through a 4-amino-4-deoxychorismate synthase resulting in L-glutamic acid and 4-amino-4-deoxychorismate. The latter product is then catalyzed via an aminodeoxychorismate lyase resulting in pyruvic acid, hydrogen ion and p-aminobenzoic acid.
(2-amino-4-hydroxy-7,8-dihydropteridin-6-yl)methyl diphosphate and p-aminobenzoic acid react with the help of a dihydropteroate synthase resulting in pyrophosphate and 7,8-dihydropteroic acid. This compound then reacts with L-glutamic acid through an ATP driven bifunctional folylpolyglutamate synthease / dihydrofolate synthease resulting in a 7,8-dihydrofolate monoglutamate. 7,8-dihydrofolate monoglutamate is then reduced via a NADPH mediated dihydrofolate reductase resulting in a tetrahydrofate which will continue and become a metabolite of the folate pathway
|
Metabolite
Metabolic
|
|
Showing 368361 -
368370 of 369801 pathways