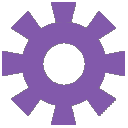
Browsing Pathways
Showing 369051 -
369060 of 605359 pathways
PathBank ID | Pathway Name and Description | Pathway Class | Chemical Compounds | Proteins |
---|---|---|---|---|
SMP0440985![]() |
Sulfur Metabolism (Isethionate)Escherichia coli O103:H2 str. 12009
The sulfur metabolism pathway starts in three possible ways. The first is the uptake of sulfate through an active transport reaction via a sulfate transport system containing an ATP-binding protein which hydrolyses ATP. Sulfate is converted by the sulfate adenylyltransferase enzymatic complex to adenosine phosphosulfate through the addition of adenine from a molecule of ATP, along with one phosphate group. Adenosine phosphosulfate is further converted to phoaphoadenosine phosphosulfate through an ATP hydrolysis and dehydrogenation reaction by the adenylyl-sulfate kinase. Phoaphoadenosine phosphosulfate is finally dehydrogenated and converted to sulfite by phosphoadenosine phosphosulfate reductase. This reaction requires magnesium, and adenosine 3',5'-diphosphate is the bi-product. A thioredoxin is also oxidized. Sulfite can also be produced from the dehydrogenation of cyanide along with the conversion of thiosulfate to thiocyanate by the thiosulfate sulfurtransferase enzymatic complex. Sulfite next undergoes a series of reactions that lead to the production of pyruvic acid, which is a precursor for pathways such as gluconeogenesis. The first reaction in this series is the conversion of sulfite to hydrogen sulfide through hygrogenation and the deoxygenation of sulfite to form a water molecule. The reaction is catalyzed by the sulfite reductase [NADPH] flavoprotein alpha and beta components. Siroheme, 4Fe-4S, flavin mononucleotide, and FAD function as cofactors or prosthetic groups. Hydrogen sulfide next undergoes dehydrogenation in a reversible reaction to form L-Cysteine and acetic acid, via the cysteine synthase complex and the coenzyme pyridoxal 5'-phosphate. L-Cysteine is dehydrogenated and converted to 2-aminoacrylic acid (a bronsted acid) and hydrogen sulfide(which may be reused) by a larger enzymatic complex composed of cysteine synthase A/B, protein malY, cystathionine-β-lyase, and tryptophanase, along with the coenzyme pyridoxal 5'-phosphate. 2-aminoacrylic acid isomerizes to 2-iminopropanoate, which along with a water molecule and a hydrogen ion is lastly converted to pyruvic acid and ammonium in a spontaneous fashion. The second possible initial starting point for sulfur metabolism is the import of taurine(an alternate sulfur source) into the cytoplasm via the taurine ABC transporter complex. Taurine, oxoglutaric acid, and oxygen are converted to sulfite by the alpha-ketoglutarate-dependent taurine dioxygenase. Carbon dioxide, succinic acid, and aminoacetaldehyde are bi-products of this reaction. Sulfite next enters pyruvic acid synthesis as already described. The third variant of sulfur metabolism starts with the import of an alkyl sulfate, in this case 1-butanesulfonate, into the cytoplasm via an aliphatic sulfonate ABC transporter complex which hydrolyses ATP. 1-butanesulfonate is dehydrogenated and along with oxygen is converted to sulfite and betaine aldehyde by the FMNH2-dependent alkanesulfonate monooxygenase enzyme. Water and flavin mononucleotide(which is used in a subsequent reaction as a prosthetic group) are also produced. Sulfite is next converted to pyruvic acid by the process already described.
|
Metabolite
Metabolic
|
|
|
SMP0440995![]() |
S-Adenosyl-L-Methionine BiosynthesisMitsuokella multacida DSM 20544
S-adenosyl-L-methionine biosynthesis(SAM) is synthesized in the cytosol of the cell from L-methionine and ATP. This reaction is catalyzed by methionine adenosyltransferase. L methione is taken up from the environment through a complex reaction coupled transport and then proceeds too synthesize the s adenosylmethionine through a adenosylmethionine synthase. S-adenosylmethionine then interacts with a hydrogen ion through an adenosylmethionine decarboxylase resulting in a carbon dioxide and a S-adenosyl 3-methioninamine. This compound interacts with a putrescine through a spermidine synthase resulting in a spermidine, a hydrogen ion and a S-methyl-5'-thioadenosine. The latter compound is degraded by interacting with a water molecule through a 5' methylthioadenosine nucleosidase resulting in an adenine and a S-methylthioribose which is then release into the environment
|
Metabolite
Metabolic
|
|
|
SMP0336804 |
Cardiolipin Biosynthesis CL(22:5(4Z,7Z,10Z,13Z,16Z)/20:4(5Z,8Z,11Z,14Z)/18:2(9Z,12Z)/16:1(9Z))Mus musculus
Cardiolipin (CL) is an important component of the inner mitochondrial membrane where it constitutes about 20% of the total lipid composition. It is essential for the optimal function of numerous enzymes that are involved in mitochondrial energy metabolism (Wikipedia). Cardiolipin biosynthesis occurs mainly in the mitochondria, but there also exists an alternative synthesis route for CDP-diacylglycerol that takes place in the endoplasmic reticulum. This second route may supplement this pathway. All membrane-localized enzymes are coloured dark green in the image. First, dihydroxyacetone phosphate (or glycerone phosphate) from glycolysis is used by the cytosolic enzyme glycerol-3-phosphate dehydrogenase [NAD(+)] to synthesize sn-glycerol 3-phosphate. Second, the mitochondrial outer membrane enzyme glycerol-3-phosphate acyltransferase esterifies an acyl-group to the sn-1 position of sn-glycerol 3-phosphate to form 1-acyl-sn-glycerol 3-phosphate (lysophosphatidic acid or LPA). Third, the enzyme 1-acyl-sn-glycerol-3-phosphate acyltransferase converts LPA into phosphatidic acid (PA or 1,2-diacyl-sn-glycerol 3-phosphate) by esterifying an acyl-group to the sn-2 position of the glycerol backbone. PA is then transferred to the inner mitochondrial membrane to continue cardiolipin synthesis. Fourth, magnesium-dependent phosphatidate cytidylyltransferase catalyzes the conversion of PA into CDP-diacylglycerol. Fifth, CDP-diacylglycerol--glycerol-3-phosphate 3-phosphatidyltransferase synthesizes phosphatidylglycerophosphate (PGP). Sixth, phosphatidylglycerophosphatase and protein-tyrosine phosphatase dephosphorylates PGP to form phosphatidylglycerol (PG). Last, cardiolipin synthase catalyzes the synthesis of cardiolipin by transferring a phosphatidyl group from a second CDP-diacylglycerol to PG. It requires a divalent metal cation cofactor.
|
Metabolite
Metabolic
|
|
|
SMP0336816 |
Cardiolipin Biosynthesis CL(22:5(4Z,7Z,10Z,13Z,16Z)/20:4(5Z,8Z,11Z,14Z)/18:4(6Z,9Z,12Z,15Z)/14:0)Mus musculus
Cardiolipin (CL) is an important component of the inner mitochondrial membrane where it constitutes about 20% of the total lipid composition. It is essential for the optimal function of numerous enzymes that are involved in mitochondrial energy metabolism (Wikipedia). Cardiolipin biosynthesis occurs mainly in the mitochondria, but there also exists an alternative synthesis route for CDP-diacylglycerol that takes place in the endoplasmic reticulum. This second route may supplement this pathway. All membrane-localized enzymes are coloured dark green in the image. First, dihydroxyacetone phosphate (or glycerone phosphate) from glycolysis is used by the cytosolic enzyme glycerol-3-phosphate dehydrogenase [NAD(+)] to synthesize sn-glycerol 3-phosphate. Second, the mitochondrial outer membrane enzyme glycerol-3-phosphate acyltransferase esterifies an acyl-group to the sn-1 position of sn-glycerol 3-phosphate to form 1-acyl-sn-glycerol 3-phosphate (lysophosphatidic acid or LPA). Third, the enzyme 1-acyl-sn-glycerol-3-phosphate acyltransferase converts LPA into phosphatidic acid (PA or 1,2-diacyl-sn-glycerol 3-phosphate) by esterifying an acyl-group to the sn-2 position of the glycerol backbone. PA is then transferred to the inner mitochondrial membrane to continue cardiolipin synthesis. Fourth, magnesium-dependent phosphatidate cytidylyltransferase catalyzes the conversion of PA into CDP-diacylglycerol. Fifth, CDP-diacylglycerol--glycerol-3-phosphate 3-phosphatidyltransferase synthesizes phosphatidylglycerophosphate (PGP). Sixth, phosphatidylglycerophosphatase and protein-tyrosine phosphatase dephosphorylates PGP to form phosphatidylglycerol (PG). Last, cardiolipin synthase catalyzes the synthesis of cardiolipin by transferring a phosphatidyl group from a second CDP-diacylglycerol to PG. It requires a divalent metal cation cofactor.
|
Metabolite
Metabolic
|
|
|
SMP0336809 |
Cardiolipin Biosynthesis CL(22:5(4Z,7Z,10Z,13Z,16Z)/20:4(5Z,8Z,11Z,14Z)/18:2(9Z,12Z)/20:2(11Z,14Z))Mus musculus
Cardiolipin (CL) is an important component of the inner mitochondrial membrane where it constitutes about 20% of the total lipid composition. It is essential for the optimal function of numerous enzymes that are involved in mitochondrial energy metabolism (Wikipedia). Cardiolipin biosynthesis occurs mainly in the mitochondria, but there also exists an alternative synthesis route for CDP-diacylglycerol that takes place in the endoplasmic reticulum. This second route may supplement this pathway. All membrane-localized enzymes are coloured dark green in the image. First, dihydroxyacetone phosphate (or glycerone phosphate) from glycolysis is used by the cytosolic enzyme glycerol-3-phosphate dehydrogenase [NAD(+)] to synthesize sn-glycerol 3-phosphate. Second, the mitochondrial outer membrane enzyme glycerol-3-phosphate acyltransferase esterifies an acyl-group to the sn-1 position of sn-glycerol 3-phosphate to form 1-acyl-sn-glycerol 3-phosphate (lysophosphatidic acid or LPA). Third, the enzyme 1-acyl-sn-glycerol-3-phosphate acyltransferase converts LPA into phosphatidic acid (PA or 1,2-diacyl-sn-glycerol 3-phosphate) by esterifying an acyl-group to the sn-2 position of the glycerol backbone. PA is then transferred to the inner mitochondrial membrane to continue cardiolipin synthesis. Fourth, magnesium-dependent phosphatidate cytidylyltransferase catalyzes the conversion of PA into CDP-diacylglycerol. Fifth, CDP-diacylglycerol--glycerol-3-phosphate 3-phosphatidyltransferase synthesizes phosphatidylglycerophosphate (PGP). Sixth, phosphatidylglycerophosphatase and protein-tyrosine phosphatase dephosphorylates PGP to form phosphatidylglycerol (PG). Last, cardiolipin synthase catalyzes the synthesis of cardiolipin by transferring a phosphatidyl group from a second CDP-diacylglycerol to PG. It requires a divalent metal cation cofactor.
|
Metabolite
Metabolic
|
|
|
SMP0441091![]() |
Sulfur MetabolismHaemophilus influenzae 86-028NP
The sulfur metabolism pathway starts in three possible ways. The first is the uptake of sulfate through an active transport reaction via a sulfate transport system containing an ATP-binding protein which hydrolyses ATP. Sulfate is converted by the sulfate adenylyltransferase enzymatic complex to adenosine phosphosulfate through the addition of adenine from a molecule of ATP, along with one phosphate group. Adenosine phosphosulfate is further converted to phoaphoadenosine phosphosulfate through an ATP hydrolysis and dehydrogenation reaction by the adenylyl-sulfate kinase. Phoaphoadenosine phosphosulfate is finally dehydrogenated and converted to sulfite by phosphoadenosine phosphosulfate reductase. This reaction requires magnesium, and adenosine 3',5'-diphosphate is the bi-product. A thioredoxin is also oxidized. Sulfite can also be produced from the dehydrogenation of cyanide along with the conversion of thiosulfate to thiocyanate by the thiosulfate sulfurtransferase enzymatic complex. Sulfite next undergoes a series of reactions that lead to the production of pyruvic acid, which is a precursor for pathways such as gluconeogenesis. The first reaction in this series is the conversion of sulfite to hydrogen sulfide through hygrogenation and the deoxygenation of sulfite to form a water molecule. The reaction is catalyzed by the sulfite reductase [NADPH] flavoprotein alpha and beta components. Siroheme, 4Fe-4S, flavin mononucleotide, and FAD function as cofactors or prosthetic groups. Hydrogen sulfide next undergoes dehydrogenation in a reversible reaction to form L-Cysteine and acetic acid, via the cysteine synthase complex and the coenzyme pyridoxal 5'-phosphate. L-Cysteine is dehydrogenated and converted to 2-aminoacrylic acid (a bronsted acid) and hydrogen sulfide(which may be reused) by a larger enzymatic complex composed of cysteine synthase A/B, protein malY, cystathionine-β-lyase, and tryptophanase, along with the coenzyme pyridoxal 5'-phosphate. 2-aminoacrylic acid isomerizes to 2-iminopropanoate, which along with a water molecule and a hydrogen ion is lastly converted to pyruvic acid and ammonium in a spontaneous fashion.
The second possible initial starting point for sulfur metabolism is the import of taurine(an alternate sulfur source) into the cytoplasm via the taurine ABC transporter complex. Taurine, oxoglutaric acid, and oxygen are converted to sulfite by the alpha-ketoglutarate-dependent taurine dioxygenase. Carbon dioxide, succinic acid, and aminoacetaldehyde are bi-products of this reaction. Sulfite next enters pyruvic acid synthesis as already described.
The third variant of sulfur metabolism starts with the import of an alkyl sulfate into the cytoplasm via an aliphatic sulfonate ABC transporter complex which hydrolyses ATP. The alkyl sulfate is dehydrogenated and along with oxygen is converted to sulfite and an aldehyde by the FMNH2-dependent alkanesulfonate monooxygenase enzyme. Water and flavin mononucleotide(which is used in a subsequent reaction as a prosthetic group) are also produced. Sulfite is next converted to pyruvic acid by the process already described.
|
Metabolite
Metabolic
|
|
|
SMP0441089![]() |
Amino Sugar and Nucleotide Sugar Metabolism IIIHalococcus morrhuae DSM 1307
The synthesis of amino sugars and nucleotide sugars starts with the phosphorylation of N-Acetylmuramic acid (MurNac) through its transport from the periplasmic space to the cytoplasm. Once in the cytoplasm, MurNac and water undergo a reversible reaction catalyzed by N-acetylmuramic acid 6-phosphate etherase, producing a D-lactic acid and N-Acetyl-D-Glucosamine 6-phosphate. This latter compound can also be introduced into the cytoplasm through a phosphorylating PTS permase in the inner membrane that allows for the transport of N-Acetyl-D-glucosamine from the periplasmic space. N-Acetyl-D-Glucosamine 6-phosphate can also be obtained from chitin dependent reactions. Chitin is hydrated through a bifunctional chitinase to produce chitobiose. This in turn gets hydrated by a beta-hexosaminidase to produce N-acetyl-D-glucosamine. The latter undergoes an atp dependent phosphorylation leading to the production of N-Acetyl-D-Glucosamine 6-phosphate. N-Acetyl-D-Glucosamine 6-phosphate is then be deacetylated in order to produce Glucosamine 6-phosphate through a N-acetylglucosamine-6-phosphate deacetylase. This compound can either be isomerized or deaminated into Beta-D-fructofuranose 6-phosphate through a glucosamine-fructose-6-phosphate aminotransferase and a glucosamine-6-phosphate deaminase respectively.
Glucosamine 6-phosphate undergoes a reversible reaction to glucosamine 1 phosphate through a phosphoglucosamine mutase. This compound is then acetylated through a bifunctional protein glmU to produce a N-Acetyl glucosamine 1-phosphate.
N-Acetyl glucosamine 1-phosphate enters the nucleotide sugar synthesis by reacting with UTP and hydrogen ion through a bifunctional protein glmU releasing pyrophosphate and a Uridine diphosphate-N-acetylglucosamine.This compound can either be isomerized into a UDP-N-acetyl-D-mannosamine or undergo a reaction with phosphoenolpyruvic acid through UDP-N-acetylglucosamine 1-carboxyvinyltransferase releasing a phosphate and a UDP-N-Acetyl-alpha-D-glucosamine-enolpyruvate.
UDP-N-acetyl-D-mannosamine undergoes a NAD dependent dehydrogenation through a UDP-N-acetyl-D-mannosamine dehydrogenase, releasing NADH, a hydrogen ion and a UDP-N-Acetyl-alpha-D-mannosaminuronate, This compound is then used in the production of enterobacterial common antigens.
UDP-N-Acetyl-alpha-D-glucosamine-enolpyruvate is reduced through a NADPH dependent UDP-N-acetylenolpyruvoylglucosamine reductase, releasing a NADP and a UDP-N-acetyl-alpha-D-muramate. This compound is involved in the D-glutamine and D-glutamate metabolism.
|
Metabolite
Metabolic
|
|
|
SMP0441084![]() |
Glycerol MetabolismHaemophilus influenzae 86-028NP
Glycerol metabolism starts with glycerol is introduced into the cytoplasm through a glycerol channel GlpF Glycerol is then phosphorylated through an ATP mediated glycerol kinase resulting in a Glycerol 3-phosphate. This compound can also be obtained through a glycerophosphodiester reacting with water through a glycerophosphoryl diester phosphodiesterase or it can also be introduced into the cytoplasm through a glycerol-3-phosphate:phosphate antiporter.
Glycerol 3-phosphate is then metabolized into a dihydroxyacetone phosphate in both aerobic or anaerobic conditions. In anaerobic conditions the metabolism is done through the reaction of glycerol 3-phosphate with a menaquinone mediated by a glycerol-3-phosphate dehydrogenase protein complex. In aerobic conditions, the metabolism is done through the reaction of glycerol 3-phosphate with ubiquinone mediated by a glycerol-3-phosphate dehydrogenase [NAD(P]+].
Dihydroxyacetone phosphate is then introduced into the fructose metabolism by turning a dihydroxyacetone into an isomer through a triosephosphate isomerase resulting in a D-glyceraldehyde 3-phosphate which in turn reacts with a phosphate through a NAD dependent Glyceraldehyde 3-phosphate dehydrogenase resulting in a glyceric acid 1,3-biphosphate. This compound is desphosphorylated by a phosphoglycerate kinase resulting in a 3-phosphoglyceric acid.This compound in turn can either react with a 2,3-bisphosphoglycerate-independent phosphoglycerate mutase or a 2,3-bisphosphoglycerate-independent phosphoglycerate mutase resulting in a 2-phospho-D-glyceric acid. This compound interacts with an enolase resulting in a phosphoenolpyruvic acid and water. Phosphoenolpyruvic acid can react either through a AMP driven phosphoenoylpyruvate synthase or a ADP driven pyruvate kinase protein complex resulting in a pyruvic acid. Pyruvic acid reacts with CoA through a NAD driven pyruvate dehydrogenase complex resulting in a carbon dioxide and a Acetyl-CoA which gets incorporated into the TCA cycle pathway.
|
Metabolite
Metabolic
|
|
|
SMP0336879 |
Cardiolipin Biosynthesis CL(22:5(4Z,7Z,10Z,13Z,16Z)/20:4(5Z,8Z,11Z,14Z)/20:5(5Z,8Z,11Z,14Z,17Z)/20:2(11Z,14Z))Mus musculus
Cardiolipin (CL) is an important component of the inner mitochondrial membrane where it constitutes about 20% of the total lipid composition. It is essential for the optimal function of numerous enzymes that are involved in mitochondrial energy metabolism (Wikipedia). Cardiolipin biosynthesis occurs mainly in the mitochondria, but there also exists an alternative synthesis route for CDP-diacylglycerol that takes place in the endoplasmic reticulum. This second route may supplement this pathway. All membrane-localized enzymes are coloured dark green in the image. First, dihydroxyacetone phosphate (or glycerone phosphate) from glycolysis is used by the cytosolic enzyme glycerol-3-phosphate dehydrogenase [NAD(+)] to synthesize sn-glycerol 3-phosphate. Second, the mitochondrial outer membrane enzyme glycerol-3-phosphate acyltransferase esterifies an acyl-group to the sn-1 position of sn-glycerol 3-phosphate to form 1-acyl-sn-glycerol 3-phosphate (lysophosphatidic acid or LPA). Third, the enzyme 1-acyl-sn-glycerol-3-phosphate acyltransferase converts LPA into phosphatidic acid (PA or 1,2-diacyl-sn-glycerol 3-phosphate) by esterifying an acyl-group to the sn-2 position of the glycerol backbone. PA is then transferred to the inner mitochondrial membrane to continue cardiolipin synthesis. Fourth, magnesium-dependent phosphatidate cytidylyltransferase catalyzes the conversion of PA into CDP-diacylglycerol. Fifth, CDP-diacylglycerol--glycerol-3-phosphate 3-phosphatidyltransferase synthesizes phosphatidylglycerophosphate (PGP). Sixth, phosphatidylglycerophosphatase and protein-tyrosine phosphatase dephosphorylates PGP to form phosphatidylglycerol (PG). Last, cardiolipin synthase catalyzes the synthesis of cardiolipin by transferring a phosphatidyl group from a second CDP-diacylglycerol to PG. It requires a divalent metal cation cofactor.
|
Metabolite
Metabolic
|
|
|
SMP0336886 |
Cardiolipin Biosynthesis CL(22:5(4Z,7Z,10Z,13Z,16Z)/20:4(5Z,8Z,11Z,14Z)/22:5(4Z,7Z,10Z,13Z,16Z)/14:0)Mus musculus
Cardiolipin (CL) is an important component of the inner mitochondrial membrane where it constitutes about 20% of the total lipid composition. It is essential for the optimal function of numerous enzymes that are involved in mitochondrial energy metabolism (Wikipedia). Cardiolipin biosynthesis occurs mainly in the mitochondria, but there also exists an alternative synthesis route for CDP-diacylglycerol that takes place in the endoplasmic reticulum. This second route may supplement this pathway. All membrane-localized enzymes are coloured dark green in the image. First, dihydroxyacetone phosphate (or glycerone phosphate) from glycolysis is used by the cytosolic enzyme glycerol-3-phosphate dehydrogenase [NAD(+)] to synthesize sn-glycerol 3-phosphate. Second, the mitochondrial outer membrane enzyme glycerol-3-phosphate acyltransferase esterifies an acyl-group to the sn-1 position of sn-glycerol 3-phosphate to form 1-acyl-sn-glycerol 3-phosphate (lysophosphatidic acid or LPA). Third, the enzyme 1-acyl-sn-glycerol-3-phosphate acyltransferase converts LPA into phosphatidic acid (PA or 1,2-diacyl-sn-glycerol 3-phosphate) by esterifying an acyl-group to the sn-2 position of the glycerol backbone. PA is then transferred to the inner mitochondrial membrane to continue cardiolipin synthesis. Fourth, magnesium-dependent phosphatidate cytidylyltransferase catalyzes the conversion of PA into CDP-diacylglycerol. Fifth, CDP-diacylglycerol--glycerol-3-phosphate 3-phosphatidyltransferase synthesizes phosphatidylglycerophosphate (PGP). Sixth, phosphatidylglycerophosphatase and protein-tyrosine phosphatase dephosphorylates PGP to form phosphatidylglycerol (PG). Last, cardiolipin synthase catalyzes the synthesis of cardiolipin by transferring a phosphatidyl group from a second CDP-diacylglycerol to PG. It requires a divalent metal cation cofactor.
|
Metabolite
Metabolic
|
|
Showing 369051 -
369060 of 369453 pathways