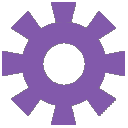
Browsing Pathways
Showing 370181 -
370190 of 605359 pathways
PathBank ID | Pathway Name and Description | Pathway Class | Chemical Compounds | Proteins |
---|---|---|---|---|
SMP0341100 |
Cardiolipin Biosynthesis CL(22:5(7Z,10Z,13Z,16Z,19Z)/22:5(4Z,7Z,10Z,13Z,16Z)/20:4(5Z,8Z,11Z,14Z)/20:2(11Z,14Z))Mus musculus
Cardiolipin (CL) is an important component of the inner mitochondrial membrane where it constitutes about 20% of the total lipid composition. It is essential for the optimal function of numerous enzymes that are involved in mitochondrial energy metabolism (Wikipedia). Cardiolipin biosynthesis occurs mainly in the mitochondria, but there also exists an alternative synthesis route for CDP-diacylglycerol that takes place in the endoplasmic reticulum. This second route may supplement this pathway. All membrane-localized enzymes are coloured dark green in the image. First, dihydroxyacetone phosphate (or glycerone phosphate) from glycolysis is used by the cytosolic enzyme glycerol-3-phosphate dehydrogenase [NAD(+)] to synthesize sn-glycerol 3-phosphate. Second, the mitochondrial outer membrane enzyme glycerol-3-phosphate acyltransferase esterifies an acyl-group to the sn-1 position of sn-glycerol 3-phosphate to form 1-acyl-sn-glycerol 3-phosphate (lysophosphatidic acid or LPA). Third, the enzyme 1-acyl-sn-glycerol-3-phosphate acyltransferase converts LPA into phosphatidic acid (PA or 1,2-diacyl-sn-glycerol 3-phosphate) by esterifying an acyl-group to the sn-2 position of the glycerol backbone. PA is then transferred to the inner mitochondrial membrane to continue cardiolipin synthesis. Fourth, magnesium-dependent phosphatidate cytidylyltransferase catalyzes the conversion of PA into CDP-diacylglycerol. Fifth, CDP-diacylglycerol--glycerol-3-phosphate 3-phosphatidyltransferase synthesizes phosphatidylglycerophosphate (PGP). Sixth, phosphatidylglycerophosphatase and protein-tyrosine phosphatase dephosphorylates PGP to form phosphatidylglycerol (PG). Last, cardiolipin synthase catalyzes the synthesis of cardiolipin by transferring a phosphatidyl group from a second CDP-diacylglycerol to PG. It requires a divalent metal cation cofactor.
|
Metabolite
Metabolic
|
|
|
SMP0341095 |
Cardiolipin Biosynthesis CL(22:5(7Z,10Z,13Z,16Z,19Z)/22:5(4Z,7Z,10Z,13Z,16Z)/20:4(5Z,8Z,11Z,14Z)/16:1(9Z))Mus musculus
Cardiolipin (CL) is an important component of the inner mitochondrial membrane where it constitutes about 20% of the total lipid composition. It is essential for the optimal function of numerous enzymes that are involved in mitochondrial energy metabolism (Wikipedia). Cardiolipin biosynthesis occurs mainly in the mitochondria, but there also exists an alternative synthesis route for CDP-diacylglycerol that takes place in the endoplasmic reticulum. This second route may supplement this pathway. All membrane-localized enzymes are coloured dark green in the image. First, dihydroxyacetone phosphate (or glycerone phosphate) from glycolysis is used by the cytosolic enzyme glycerol-3-phosphate dehydrogenase [NAD(+)] to synthesize sn-glycerol 3-phosphate. Second, the mitochondrial outer membrane enzyme glycerol-3-phosphate acyltransferase esterifies an acyl-group to the sn-1 position of sn-glycerol 3-phosphate to form 1-acyl-sn-glycerol 3-phosphate (lysophosphatidic acid or LPA). Third, the enzyme 1-acyl-sn-glycerol-3-phosphate acyltransferase converts LPA into phosphatidic acid (PA or 1,2-diacyl-sn-glycerol 3-phosphate) by esterifying an acyl-group to the sn-2 position of the glycerol backbone. PA is then transferred to the inner mitochondrial membrane to continue cardiolipin synthesis. Fourth, magnesium-dependent phosphatidate cytidylyltransferase catalyzes the conversion of PA into CDP-diacylglycerol. Fifth, CDP-diacylglycerol--glycerol-3-phosphate 3-phosphatidyltransferase synthesizes phosphatidylglycerophosphate (PGP). Sixth, phosphatidylglycerophosphatase and protein-tyrosine phosphatase dephosphorylates PGP to form phosphatidylglycerol (PG). Last, cardiolipin synthase catalyzes the synthesis of cardiolipin by transferring a phosphatidyl group from a second CDP-diacylglycerol to PG. It requires a divalent metal cation cofactor.
|
Metabolite
Metabolic
|
|
|
SMP0425411![]() |
Oxidative Phosphorylation[Bacteroides] pectinophilus ATCC 43243
The process of oxidative phosphorylation involves multiple interactions of ubiquinone with succinic acid, resulting in a fumaric acid and ubiquinol.
Ubiquinone interacts with succinic acid through a succinate:quinone oxidoreductase resulting in a fumaric acid an ubiquinol. This enzyme has various cofactors, ferroheme b, 2FE-2S, FAD, and 3Fe-4S iron-sulfur cluster.
Then 2 ubiquinol interact with oxygen and 4 hydrogen ion through a cytochrome bd-I terminal oxidase resulting in a 4 hydrogen ion transferred into the periplasmic space, 2 water returned into the cytoplasm and 2 ubiquinone, which stay in the inner membrane.
The ubiquinone interacts with succinic acid through a succinate:quinone oxidoreductase resulting in a fumaric acid an ubiquinol.
Then 2 ubiquinol interacts with oxygen and 4 hydrogen ion through a cytochrome bd-II terminal oxidase resulting in a 4 hydrogen ion transferred into the periplasmic space, 2 water returned into the cytoplasm and 2 ubiquinone, which stay in the inner membrane.
The ubiquinone interacts with succinic acid through a succinate:quinone oxidoreductase resulting in a fumaric acid an ubiquinol.
The 2 ubiquinol interact with oxygen and 8 hydrogen ion through a cytochrome bo terminal oxidase resulting in a 8 hydrogen ion transferred into the periplasmic space, 2 water returned into the cytoplasm and 2 ubiquinone, which stays in the inner membrane.
The ubiquinone then interacts with 5 hydrogen ion through a NADH dependent ubiquinone oxidoreductase I resulting in NAD, hydrogen ion released into the periplasmic space and an ubiquinol.
The ubiquinol is then processed reacting with oxygen, and 4 hydrogen through a ion cytochrome bd-I terminal oxidase resulting in 4 hydrogen ions released into the periplasmic space, 2 water molecules into the cytoplasm and 2 ubiquinones.
The ubiquinone then interacts with 5 hydrogen ion through a NADH dependent ubiquinone oxidoreductase I resulting in NAD, hydrogen ion released into the periplasmic space and an ubiquinol.
The 2 ubiquinol interact with oxygen and 8 hydrogen ion through a cytochrome bo terminal oxidase resulting in a 8 hydrogen ion transferred into the periplasmic space, 2 water returned into the cytoplasm and 2 ubiquinone, which stays in the inner membrane.
|
Metabolite
Metabolic
|
|
|
SMP0445964![]() |
Porphyrin MetabolismMitsuokella multacida DSM 20544
The metabolism of porphyrin begins with with glutamic acid being processed by an ATP-driven glutamyl-tRNA synthetase by interacting with hydrogen ion and tRNA(Glu), resulting in amo, pyrophosphate and L-glutamyl-tRNA(Glu) Glutamic acid. Glutamic acid can be obtained as a result of L-glutamate metabolism pathway, glutamate / aspartate : H+ symporter GltP, glutamate:sodium symporter or a glutamate / aspartate ABC transporter .
L-glutamyl-tRNA(Glu) Glutamic acid interacts with a NADPH glutamyl-tRNA reductase resulting in a NADP, a tRNA(Glu) and a (S)-4-amino-5-oxopentanoate.
This compound interacts with a glutamate-1-semialdehyde aminotransferase resulting a 5-aminolevulinic acid. This compound interacts with a porphobilinogen synthase resulting in a hydrogen ion, water and porphobilinogen. The latter compound interacts with water resulting in hydroxymethylbilane synthase resulting in ammonium, and hydroxymethylbilane.
Hydroxymethylbilane can either be dehydrated to produce uroporphyrinogen I or interact with a uroporphyrinogen III synthase resulting in a water molecule and a uroporphyrinogen III.
Uroporphyrinogen I interacts with hydrogen ion through a uroporphyrinogen decarboxylase resulting in a carbon dioxide and a coproporphyrinogen I
Uroporphyrinogen III can be metabolized into precorrin by interacting with a S-adenosylmethionine through a siroheme synthase resulting in hydrogen ion, an s-adenosylhomocysteine and a precorrin-1. On the other hand, Uroporphyrinogen III interacts with hydrogen ion through a uroporphyrinogen decarboxylase resulting in a carbon dioxide and a Coproporphyrinogen III.
Precorrin-1 reacts with a S-adenosylmethionine through a siroheme synthase resulting in a S-adenosylhomocysteine and a Precorrin-2. The latter compound is processed by a NAD dependent uroporphyrin III C-methyltransferase [multifunctional] resulting in a NADH and a sirohydrochlorin. This compound then interacts with Fe 2+
uroporphyrin III C-methyltransferase [multifunctional] resulting in a hydrogen ion and a siroheme. The siroheme is then processed in sulfur metabolism pathway.
Uroporphyrinogen III can be processed in anaerobic or aerobic condition.
Anaerobic:
Uroporphyrinogen III interacts with an oxygen molecule, a hydrogen ion through a coproporphyrinogen III oxidase resulting in water, carbon dioxide and protoporphyrinogen IX. The latter compound then interacts with an 3 oxygen molecule through a protoporphyrinogen oxidase resulting in 3 hydrogen peroxide and a Protoporphyrin IX
Aerobic:
Uroporphyrinogen III reacts with S-adenosylmethionine through a coproporphyrinogen III dehydrogenase resulting in carbon dioxide, 5-deoxyadenosine, L-methionine and protoporphyrinogen IX. The latter compound interacts with a meanquinone through a protoporphyrinogen oxidase resulting in protoporphyrin IX.
The protoporphyrin IX interacts with Fe 2+ through a ferrochelatase resulting in a hydrogen ion and a ferroheme b. The ferroheme b can either be incorporated into the oxidative phosphorylation as a cofactor of the enzymes involved in that pathway or it can interact with hydrogen peroxide through a catalase HPII resulting in a heme D. Heme D can then be incorporated into the oxidative phosphyrlation pathway as a cofactor of the enzymes involved in that pathway. Ferroheme b can also interact with water and a farnesyl pyrophosphate through a heme O synthase resulting in a release of pyrophosphate and heme O. Heme O is then incorporated into the Oxidative phosphorylation pathway.
|
Metabolite
Metabolic
|
|
|
SMP0445947![]() |
Secondary Metabolites: Enterobacterial Common Antigen BiosynthesisProteus mirabilis HI4320
The biosynthesis of a enterobacterial common antigen can begin with a di-trans,octa-cis-undecaprenyl phosphate interacts with a Uridine diphosphate-N-acetylglucosamine through undecaprenyl-phosphate α-N-acetylglucosaminyl transferase resulting in a N-acetyl-α-D-glucosaminyl-diphospho-ditrans,octacis-undecaprenol and a Uridine 5'-monophosphate. The N-acetyl-α-D-glucosaminyl-diphospho-ditrans,octacis-undecaprenol then reacts with an UDP-ManNAcA from the Amino sugar and nucleotide sugar metabolism pathway. This reaction is metabolized by a UDP-N-acetyl-D-mannosaminuronic acid transferase resulting in a uridine 5' diphosphate, a hydrogen ion and a Undecaprenyl N-acetyl-glucosaminyl-N-acetyl-mannosaminuronate-4-acetamido-4,6-dideoxy-D-galactose pyrophosphate. Glucose 1 phosphate can be metabolize by interacting with a hydrogen ion and a thymidine 5-triphosphate by either reacting with a dTDP-glucose pyrophosphorylase or a dTDP-glucose pyrophosphorylase 2 resulting in the release of a pyrophosphate and a dTDP-D-glucose. The latter compound is then dehydrated through an dTDP-glucose 4,6-dehydratase 2 resulting in water and dTDP-4-dehydro-6-deoxy-D-glucose. The latter compound interacts with L-glutamic acid through a dTDP-4-dehydro-6-deoxy-D-glucose transaminase resulting in the release of oxoglutaric acid and dTDP-thomosamine. The latter compound interacts with acetyl-coa through a dTDP-fucosamine acetyltransferase resulting in a Coenzyme A, a hydrogen Ion and a TDP-Fuc4NAc. Undecaprenyl N-acetyl-glucosaminyl-N-acetyl-mannosaminuronate-4-acetamido-4,6-dideoxy-D-galactose pyrophosphate then interacts with a TDP--Fuc4NAc through a 4-acetamido-4,6-dideoxy-D-galactose transferase resulting in a hydrogen ion, a dTDP and a Undecaprenyl N-acetyl-glucosaminyl-N-acetyl-mannosaminuronate-4-acetamido-4,6-dideoxy-D-galactose pyrophosphate. This compound is then transported through a protein wzxE into the periplasmic space so that it can be incorporated into the outer membrane.
|
Metabolite
Metabolic
|
|
|
SMP0341165 |
Cardiolipin Biosynthesis CL(22:6(4Z,7Z,10Z,13Z,16Z,19Z)/22:5(4Z,7Z,10Z,13Z,16Z)/16:0/16:0)Mus musculus
Cardiolipin (CL) is an important component of the inner mitochondrial membrane where it constitutes about 20% of the total lipid composition. It is essential for the optimal function of numerous enzymes that are involved in mitochondrial energy metabolism (Wikipedia). Cardiolipin biosynthesis occurs mainly in the mitochondria, but there also exists an alternative synthesis route for CDP-diacylglycerol that takes place in the endoplasmic reticulum. This second route may supplement this pathway. All membrane-localized enzymes are coloured dark green in the image. First, dihydroxyacetone phosphate (or glycerone phosphate) from glycolysis is used by the cytosolic enzyme glycerol-3-phosphate dehydrogenase [NAD(+)] to synthesize sn-glycerol 3-phosphate. Second, the mitochondrial outer membrane enzyme glycerol-3-phosphate acyltransferase esterifies an acyl-group to the sn-1 position of sn-glycerol 3-phosphate to form 1-acyl-sn-glycerol 3-phosphate (lysophosphatidic acid or LPA). Third, the enzyme 1-acyl-sn-glycerol-3-phosphate acyltransferase converts LPA into phosphatidic acid (PA or 1,2-diacyl-sn-glycerol 3-phosphate) by esterifying an acyl-group to the sn-2 position of the glycerol backbone. PA is then transferred to the inner mitochondrial membrane to continue cardiolipin synthesis. Fourth, magnesium-dependent phosphatidate cytidylyltransferase catalyzes the conversion of PA into CDP-diacylglycerol. Fifth, CDP-diacylglycerol--glycerol-3-phosphate 3-phosphatidyltransferase synthesizes phosphatidylglycerophosphate (PGP). Sixth, phosphatidylglycerophosphatase and protein-tyrosine phosphatase dephosphorylates PGP to form phosphatidylglycerol (PG). Last, cardiolipin synthase catalyzes the synthesis of cardiolipin by transferring a phosphatidyl group from a second CDP-diacylglycerol to PG. It requires a divalent metal cation cofactor.
|
Metabolite
Metabolic
|
|
|
SMP0341157 |
Cardiolipin Biosynthesis CL(22:6(4Z,7Z,10Z,13Z,16Z,19Z)/22:5(4Z,7Z,10Z,13Z,16Z)/14:0/20:2(11Z,14Z))Mus musculus
Cardiolipin (CL) is an important component of the inner mitochondrial membrane where it constitutes about 20% of the total lipid composition. It is essential for the optimal function of numerous enzymes that are involved in mitochondrial energy metabolism (Wikipedia). Cardiolipin biosynthesis occurs mainly in the mitochondria, but there also exists an alternative synthesis route for CDP-diacylglycerol that takes place in the endoplasmic reticulum. This second route may supplement this pathway. All membrane-localized enzymes are coloured dark green in the image. First, dihydroxyacetone phosphate (or glycerone phosphate) from glycolysis is used by the cytosolic enzyme glycerol-3-phosphate dehydrogenase [NAD(+)] to synthesize sn-glycerol 3-phosphate. Second, the mitochondrial outer membrane enzyme glycerol-3-phosphate acyltransferase esterifies an acyl-group to the sn-1 position of sn-glycerol 3-phosphate to form 1-acyl-sn-glycerol 3-phosphate (lysophosphatidic acid or LPA). Third, the enzyme 1-acyl-sn-glycerol-3-phosphate acyltransferase converts LPA into phosphatidic acid (PA or 1,2-diacyl-sn-glycerol 3-phosphate) by esterifying an acyl-group to the sn-2 position of the glycerol backbone. PA is then transferred to the inner mitochondrial membrane to continue cardiolipin synthesis. Fourth, magnesium-dependent phosphatidate cytidylyltransferase catalyzes the conversion of PA into CDP-diacylglycerol. Fifth, CDP-diacylglycerol--glycerol-3-phosphate 3-phosphatidyltransferase synthesizes phosphatidylglycerophosphate (PGP). Sixth, phosphatidylglycerophosphatase and protein-tyrosine phosphatase dephosphorylates PGP to form phosphatidylglycerol (PG). Last, cardiolipin synthase catalyzes the synthesis of cardiolipin by transferring a phosphatidyl group from a second CDP-diacylglycerol to PG. It requires a divalent metal cation cofactor.
|
Metabolite
Metabolic
|
|
|
SMP0445953![]() |
Secondary Metabolites: Enterobacterial Common Antigen BiosynthesisCampylobacter jejuni subsp. jejuni M1
The biosynthesis of a enterobacterial common antigen can begin with a di-trans,octa-cis-undecaprenyl phosphate interacts with a Uridine diphosphate-N-acetylglucosamine through undecaprenyl-phosphate α-N-acetylglucosaminyl transferase resulting in a N-acetyl-α-D-glucosaminyl-diphospho-ditrans,octacis-undecaprenol and a Uridine 5'-monophosphate. The N-acetyl-α-D-glucosaminyl-diphospho-ditrans,octacis-undecaprenol then reacts with an UDP-ManNAcA from the Amino sugar and nucleotide sugar metabolism pathway. This reaction is metabolized by a UDP-N-acetyl-D-mannosaminuronic acid transferase resulting in a uridine 5' diphosphate, a hydrogen ion and a Undecaprenyl N-acetyl-glucosaminyl-N-acetyl-mannosaminuronate-4-acetamido-4,6-dideoxy-D-galactose pyrophosphate. Glucose 1 phosphate can be metabolize by interacting with a hydrogen ion and a thymidine 5-triphosphate by either reacting with a dTDP-glucose pyrophosphorylase or a dTDP-glucose pyrophosphorylase 2 resulting in the release of a pyrophosphate and a dTDP-D-glucose. The latter compound is then dehydrated through an dTDP-glucose 4,6-dehydratase 2 resulting in water and dTDP-4-dehydro-6-deoxy-D-glucose. The latter compound interacts with L-glutamic acid through a dTDP-4-dehydro-6-deoxy-D-glucose transaminase resulting in the release of oxoglutaric acid and dTDP-thomosamine. The latter compound interacts with acetyl-coa through a dTDP-fucosamine acetyltransferase resulting in a Coenzyme A, a hydrogen Ion and a TDP-Fuc4NAc. Undecaprenyl N-acetyl-glucosaminyl-N-acetyl-mannosaminuronate-4-acetamido-4,6-dideoxy-D-galactose pyrophosphate then interacts with a TDP--Fuc4NAc through a 4-acetamido-4,6-dideoxy-D-galactose transferase resulting in a hydrogen ion, a dTDP and a Undecaprenyl N-acetyl-glucosaminyl-N-acetyl-mannosaminuronate-4-acetamido-4,6-dideoxy-D-galactose pyrophosphate. This compound is then transported through a protein wzxE into the periplasmic space so that it can be incorporated into the outer membrane.
|
Metabolite
Metabolic
|
|
|
SMP0445959![]() |
Secondary Metabolites: Glyoxylate CycleCampylobacter jejuni subsp. jejuni M1
The glyoxylate cycle starts with the interaction of Acetyl-Coa with a water molecule and Oxalacetic acid interact through a Citrate synthase resulting in a release of a coenzyme a and citric acid. The citric acid gets dehydrated through a citrate hydro-lyase resulting in the release of a water molecule and cis-Aconitic acid. The cis-Aconitic acid is then hydrated in an reversible reaction through an aconitate hydratase resulting in an Isocitric acid. The isocitric acid then interacts in a reversible reaction through isocitrate lyase resulting in the release of a succinic acid and a glyoxylic acid. The glyoxylic acid then reacts in a reversible reaction with an acetyl-coa, and a water molecule in a reversible reaction, resulting in a release of a coenzyme A, a hydrogen ion and an L-malic acid. The L-malic acid interacts in a reversible reaction through a NAD driven malate dehydrogenase resulting in the release of NADH, a hydrogen ion and an Oxalacetic acid.
|
Metabolite
Metabolic
|
||
SMP0445970![]() |
Secondary Metabolites: Threonine Biosynthesis from AspartateEscherichia coli DH1
The biosynthesis of threonine starts with L-aspartic acid being phosphorylated by an ATP driven Aspartate kinase resulting in an a release of an ADP and an L-aspartyl-4-phosphate. This compound interacts with a hydrogen ion through an NADPH driven aspartate semialdehyde dehydrogenase resulting in the release of a phosphate, an NADP and a L-aspartate-semialdehyde.The latter compound interacts with a hydrogen ion through a NADPH driven aspartate kinase / homoserine dehydrogenase resulting in the release of an NADP and a L-homoserine. L-homoserine is phosphorylated through an ATP driven homoserine kinase resulting in the release of an ADP, a hydrogen ion and a O-phosphohomoserine. The latter compound then interacts with a water molecule threonine synthase resulting in the release of a phosphate and an L-threonine.
|
Metabolite
Metabolic
|
Showing 370181 -
370190 of 370471 pathways