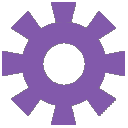
Browsing Pathways
Showing 371441 -
371450 of 605359 pathways
PathBank ID | Pathway Name and Description | Pathway Class | Chemical Compounds | Proteins |
---|---|---|---|---|
SMP0449143![]() |
Hexuronide and Hexuronate DegradationEscherichia coli O55:H7 str. CB9615
Beta-D-glucuronosides, D-glucuronate and D-fructuronate can be used as a source of carbon for E.coli. They are imported into E.coli's periplasmic space by membrane-associated protein (UidC/gusC), and are further imported into cytoplasm by hydrogen symporter. Beta-glucuronides undergoes hydrolysis by beta-D-glucuronidase to form D-glucuronate. D-glucuronate is isomerized by D-glucuronate isomerase to form D-fructuronate. D-fructuronate is further reduced to D-mannonate by D-mannonate oxidoreductase. D-mannonate dehydratase dehydrated to yield 2-dehydro-3-deoxy-D-gluconate. At this point, a common enzyme, 2-keto-3-deoxygluconokinase, phosphorylates 2-dehydro-3-deoxy-D-gluconate to yield 2-dehydro-3-deoxy-D-gluconate-6-phosphate. This product is then process by KHG/KDPG aldolase which in turn produces D-Glyceraldehyde 3-phosphate and Pyruvic Acid which then go into their respective sub pathways: glycolysis and pyruvate dehydrogenase. The pathway can also start from 3 other points: a hydrogen ion symporter (gluconate/fructuronate transporter GntP) of D-fructuronate, a hydrogen ion symporter (Hexuronate transporter) of aldehydo-D-galacturonate that spontaneously turns into D-tagaturonate. This compound can also be obtained by the reaction of aldehydo-L-galactonate with a NAD dependent l-galactonate oxidoreductase resulting in the release of NADH, hydrogen ion. Tagaturonate then undergoes an NADH-dependent reduction to D-altronate through an altronate oxidoreductase. D-altronate undergoes dehydration to yield 2-dehydro-3-deoxy-D-gluconate, the third and last point where the reaction can start from a hydrogen symporter of a 2-dehydro-3-deoy-D-gluconate.
|
Metabolite
Metabolic
|
|
|
SMP0449150![]() |
Cardiolipin Biosynthesis CL(i-15:0/i-16:0/i-18:0/i-19:0)Homo sapiens
Cardiolipin (CL) is an important component of the inner mitochondrial membrane where it constitutes about 20% of the total lipid composition. It is essential for the optimal function of numerous enzymes that are involved in mitochondrial energy metabolism (Wikipedia). Cardiolipin biosynthesis occurs mainly in the mitochondria, but there also exists an alternative synthesis route for CDP-diacylglycerol that takes place in the endoplasmic reticulum. This second route may supplement this pathway. All membrane-localized enzymes are coloured dark green in the image. First, dihydroxyacetone phosphate (or glycerone phosphate) from glycolysis is used by the cytosolic enzyme glycerol-3-phosphate dehydrogenase [NAD(+)] to synthesize sn-glycerol 3-phosphate. Second, the mitochondrial outer membrane enzyme glycerol-3-phosphate acyltransferase esterifies an acyl-group to the sn-1 position of sn-glycerol 3-phosphate to form 1-acyl-sn-glycerol 3-phosphate (lysophosphatidic acid or LPA). Third, the enzyme 1-acyl-sn-glycerol-3-phosphate acyltransferase converts LPA into phosphatidic acid (PA or 1,2-diacyl-sn-glycerol 3-phosphate) by esterifying an acyl-group to the sn-2 position of the glycerol backbone. PA is then transferred to the inner mitochondrial membrane to continue cardiolipin synthesis. Fourth, magnesium-dependent phosphatidate cytidylyltransferase catalyzes the conversion of PA into CDP-diacylglycerol. Fifth, CDP-diacylglycerol--glycerol-3-phosphate 3-phosphatidyltransferase synthesizes phosphatidylglycerophosphate (PGP). Sixth, phosphatidylglycerophosphatase and protein-tyrosine phosphatase dephosphorylates PGP to form phosphatidylglycerol (PG). Last, cardiolipin synthase catalyzes the synthesis of cardiolipin by transferring a phosphatidyl group from a second CDP-diacylglycerol to PG. It requires a divalent metal cation cofactor.
|
Metabolite
Metabolic
|
|
|
SMP0449375![]() |
Pyrimidine MetabolismSerratia marcescens subsp. marcescens Db11
The metabolism of pyrimidines begins with L-glutamine interacting with water molecule and a hydrogen carbonate through an ATP driven carbamoyl phosphate synthetase resulting in a hydrogen ion, an ADP, a phosphate, an L-glutamic acid and a carbamoyl phosphate. The latter compound interacts with an L-aspartic acid through a aspartate transcarbamylase resulting in a phosphate, a hydrogen ion and a N-carbamoyl-L-aspartate. The latter compound interacts with a hydrogen ion through a dihydroorotase resulting in the release of a water molecule and a 4,5-dihydroorotic acid. This compound interacts with an ubiquinone-1 through a dihydroorotate dehydrogenase, type 2 resulting in a release of an ubiquinol-1 and an orotic acid. The orotic acid then interacts with a phosphoribosyl pyrophosphate through a orotate phosphoribosyltransferase resulting in a pyrophosphate and an orotidylic acid. The latter compound then interacts with a hydrogen ion through an orotidine-5 '-phosphate decarboxylase, resulting in an release of carbon dioxide and an Uridine 5' monophosphate. The Uridine 5' monophosphate process to get phosphorylated by an ATP driven UMP kinase resulting in the release of an ADP and an Uridine 5--diphosphate. Uridine 5-diphosphate can be metabolized in multiple ways in order to produce a Deoxyuridine triphosphate. 1.-Uridine 5-diphosphate interacts with a reduced thioredoxin through a ribonucleoside diphosphate reductase 1 resulting in the release of a water molecule and an oxidized thioredoxin and an dUDP. The dUDP is then phosphorylated by an ATP through a nucleoside diphosphate kinase resulting in the release of an ADP and a DeoxyUridine triphosphate. 2.-Uridine 5-diphosphate interacts with a reduced NrdH glutaredoxin-like protein through a Ribonucleoside-diphosphate reductase 1 resulting in a release of a water molecule, an oxidized NrdH glutaredoxin-like protein and a dUDP. The dUDP is then phosphorylated by an ATP through a nucleoside diphosphate kinase resulting in the release of an ADP and a DeoxyUridine triphosphate. 3.-Uridine 5-diphosphate is phosphorylated by an ATP-driven nucleoside diphosphate kinase resulting in an ADP and an Uridinetriphosphate. The latter compound interacts with a reduced flavodoxin through ribonucleoside-triphosphate reductase resulting in the release of an oxidized flavodoxin, a water molecule and a Deoxyuridine triphosphate 4.-Uridine 5-diphosphate is phosphorylated by an ATP-driven nucleoside diphosphate kinase resulting in an ADP and an Uridinetriphosphate The uridine triphosphate interacts with a L-glutamine and a water molecule through an ATP driven CTP synthase resulting in an ADP, a phosphate, a hydrogen ion, an L-glutamic acid and a cytidine triphosphate. The cytidine triphosphate interacts with a reduced flavodoxin through a ribonucleoside-triphosphate reductase resulting in the release of a water molecule, an oxidized flavodoxin and a dCTP. The dCTP interacts with a water molecule and a hydrogen ion through a dCTP deaminase resulting in a release of an ammonium molecule and a Deoxyuridine triphosphate. 5.-Uridine 5-diphosphate is phosphorylated by an ATP-driven nucleoside diphosphate kinase resulting in an ADP and an Uridinetriphosphate The uridine triphosphate interacts with a L-glutamine and a water molecule through an ATP driven CTP synthase resulting in an ADP, a phosphate, a hydrogen ion, an L-glutamic acid and a cytidine triphosphate. The cytidine triphosphate then interacts spontaneously with a water molecule resulting in the release of a phosphate, a hydrogen ion and a CDP. The CDP then interacts with a reduced NrdH glutaredoxin-like protein through a ribonucleoside-diphosphate reductase 2 resulting in the release of a water molecule, an oxidized NrdH glutaredoxin-like protein and a dCDP. The dCDP is then phosphorylated through an ATP driven nucleoside diphosphate kinase resulting in an ADP and a dCTP. The dCTP interacts with a water molecule and a hydrogen ion through a dCTP deaminase resulting in a release of an ammonium molecule and a Deoxyuridine triphosphate. 6.-Uridine 5-diphosphate is phosphorylated by an ATP-driven nucleoside diphosphate kinase resulting in an ADP and an Uridinetriphosphate The uridine triphosphate interacts with a L-glutamine and a water molecule through an ATP driven CTP synthase resulting in an ADP, a phosphate, a hydrogen ion, an L-glutamic acid and a cytidine triphosphate. The cytidine triphosphate then interacts spontaneously with a water molecule resulting in the release of a phosphate, a hydrogen ion and a CDP. The CDP interacts with a reduced thioredoxin through a ribonucleoside diphosphate reductase 1 resulting in a release of a water molecule, an oxidized thioredoxin and a dCDP. The dCDP is then phosphorylated through an ATP driven nucleoside diphosphate kinase resulting in an ADP and a dCTP. The dCTP interacts with a water molecule and a hydrogen ion through a dCTP deaminase resulting in a release of an ammonium molecule and a Deoxyuridine triphosphate. The deoxyuridine triphosphate then interacts with a water molecule through a nucleoside triphosphate pyrophosphohydrolase resulting in a release of a hydrogen ion, a phosphate and a dUMP. The dUMP then interacts with a methenyltetrahydrofolate through a thymidylate synthase resulting in a dihydrofolic acid and a 5-thymidylic acid. Then 5-thymidylic acid is then phosphorylated through a nucleoside diphosphate kinase resulting in the release of an ADP and thymidine 5'-triphosphate.
|
Metabolite
Metabolic
|
|
|
SMP0449385![]() |
Glutathione MetabolismMesorhizobium loti MAFF303099
The biosynthesis of glutathione starts with the introduction of L-glutamic acid through either a glutamate:sodium symporter, glutamate / aspartate : H+ symporter GltP or a
glutamate / aspartate ABC transporter. Once in the cytoplasm, L-glutamice acid reacts with L-cysteine through an ATP glutamate-cysteine ligase resulting in gamma-glutamylcysteine. This compound reacts which Glycine through an ATP driven glutathione synthetase thus catabolizing Glutathione.
This compound is metabolized through a spontaneous reaction with an oxidized glutaredoxin resulting in a reduced glutaredoxin and an oxidized glutathione. This compound is reduced by a NADPH glutathione reductase resulting in a glutathione.
Glutathione can then be degraded into various different glutathione containing compounds by reacting with a napthalene or Bromobenzene-2,3-oxide through a glutathione S-transferase.
|
Metabolite
Metabolic
|
|
|
SMP0344016 |
Cardiolipin Biosynthesis CL(20:5(5Z,8Z,11Z,14Z,17Z)/22:6(4Z,7Z,10Z,13Z,16Z,19Z)/16:1(9Z)/18:4(6Z,9Z,12Z,15Z))Mus musculus
Cardiolipin (CL) is an important component of the inner mitochondrial membrane where it constitutes about 20% of the total lipid composition. It is essential for the optimal function of numerous enzymes that are involved in mitochondrial energy metabolism (Wikipedia). Cardiolipin biosynthesis occurs mainly in the mitochondria, but there also exists an alternative synthesis route for CDP-diacylglycerol that takes place in the endoplasmic reticulum. This second route may supplement this pathway. All membrane-localized enzymes are coloured dark green in the image. First, dihydroxyacetone phosphate (or glycerone phosphate) from glycolysis is used by the cytosolic enzyme glycerol-3-phosphate dehydrogenase [NAD(+)] to synthesize sn-glycerol 3-phosphate. Second, the mitochondrial outer membrane enzyme glycerol-3-phosphate acyltransferase esterifies an acyl-group to the sn-1 position of sn-glycerol 3-phosphate to form 1-acyl-sn-glycerol 3-phosphate (lysophosphatidic acid or LPA). Third, the enzyme 1-acyl-sn-glycerol-3-phosphate acyltransferase converts LPA into phosphatidic acid (PA or 1,2-diacyl-sn-glycerol 3-phosphate) by esterifying an acyl-group to the sn-2 position of the glycerol backbone. PA is then transferred to the inner mitochondrial membrane to continue cardiolipin synthesis. Fourth, magnesium-dependent phosphatidate cytidylyltransferase catalyzes the conversion of PA into CDP-diacylglycerol. Fifth, CDP-diacylglycerol--glycerol-3-phosphate 3-phosphatidyltransferase synthesizes phosphatidylglycerophosphate (PGP). Sixth, phosphatidylglycerophosphatase and protein-tyrosine phosphatase dephosphorylates PGP to form phosphatidylglycerol (PG). Last, cardiolipin synthase catalyzes the synthesis of cardiolipin by transferring a phosphatidyl group from a second CDP-diacylglycerol to PG. It requires a divalent metal cation cofactor.
|
Metabolite
Metabolic
|
|
|
SMP0344023 |
Cardiolipin Biosynthesis CL(20:5(5Z,8Z,11Z,14Z,17Z)/22:6(4Z,7Z,10Z,13Z,16Z,19Z)/16:1(9Z)/20:5(5Z,8Z,11Z,14Z,17Z))Mus musculus
Cardiolipin (CL) is an important component of the inner mitochondrial membrane where it constitutes about 20% of the total lipid composition. It is essential for the optimal function of numerous enzymes that are involved in mitochondrial energy metabolism (Wikipedia). Cardiolipin biosynthesis occurs mainly in the mitochondria, but there also exists an alternative synthesis route for CDP-diacylglycerol that takes place in the endoplasmic reticulum. This second route may supplement this pathway. All membrane-localized enzymes are coloured dark green in the image. First, dihydroxyacetone phosphate (or glycerone phosphate) from glycolysis is used by the cytosolic enzyme glycerol-3-phosphate dehydrogenase [NAD(+)] to synthesize sn-glycerol 3-phosphate. Second, the mitochondrial outer membrane enzyme glycerol-3-phosphate acyltransferase esterifies an acyl-group to the sn-1 position of sn-glycerol 3-phosphate to form 1-acyl-sn-glycerol 3-phosphate (lysophosphatidic acid or LPA). Third, the enzyme 1-acyl-sn-glycerol-3-phosphate acyltransferase converts LPA into phosphatidic acid (PA or 1,2-diacyl-sn-glycerol 3-phosphate) by esterifying an acyl-group to the sn-2 position of the glycerol backbone. PA is then transferred to the inner mitochondrial membrane to continue cardiolipin synthesis. Fourth, magnesium-dependent phosphatidate cytidylyltransferase catalyzes the conversion of PA into CDP-diacylglycerol. Fifth, CDP-diacylglycerol--glycerol-3-phosphate 3-phosphatidyltransferase synthesizes phosphatidylglycerophosphate (PGP). Sixth, phosphatidylglycerophosphatase and protein-tyrosine phosphatase dephosphorylates PGP to form phosphatidylglycerol (PG). Last, cardiolipin synthase catalyzes the synthesis of cardiolipin by transferring a phosphatidyl group from a second CDP-diacylglycerol to PG. It requires a divalent metal cation cofactor.
|
Metabolite
Metabolic
|
|
|
SMP0344011 |
Cardiolipin Biosynthesis CL(20:5(5Z,8Z,11Z,14Z,17Z)/22:6(4Z,7Z,10Z,13Z,16Z,19Z)/16:1(9Z)/18:0)Mus musculus
Cardiolipin (CL) is an important component of the inner mitochondrial membrane where it constitutes about 20% of the total lipid composition. It is essential for the optimal function of numerous enzymes that are involved in mitochondrial energy metabolism (Wikipedia). Cardiolipin biosynthesis occurs mainly in the mitochondria, but there also exists an alternative synthesis route for CDP-diacylglycerol that takes place in the endoplasmic reticulum. This second route may supplement this pathway. All membrane-localized enzymes are coloured dark green in the image. First, dihydroxyacetone phosphate (or glycerone phosphate) from glycolysis is used by the cytosolic enzyme glycerol-3-phosphate dehydrogenase [NAD(+)] to synthesize sn-glycerol 3-phosphate. Second, the mitochondrial outer membrane enzyme glycerol-3-phosphate acyltransferase esterifies an acyl-group to the sn-1 position of sn-glycerol 3-phosphate to form 1-acyl-sn-glycerol 3-phosphate (lysophosphatidic acid or LPA). Third, the enzyme 1-acyl-sn-glycerol-3-phosphate acyltransferase converts LPA into phosphatidic acid (PA or 1,2-diacyl-sn-glycerol 3-phosphate) by esterifying an acyl-group to the sn-2 position of the glycerol backbone. PA is then transferred to the inner mitochondrial membrane to continue cardiolipin synthesis. Fourth, magnesium-dependent phosphatidate cytidylyltransferase catalyzes the conversion of PA into CDP-diacylglycerol. Fifth, CDP-diacylglycerol--glycerol-3-phosphate 3-phosphatidyltransferase synthesizes phosphatidylglycerophosphate (PGP). Sixth, phosphatidylglycerophosphatase and protein-tyrosine phosphatase dephosphorylates PGP to form phosphatidylglycerol (PG). Last, cardiolipin synthase catalyzes the synthesis of cardiolipin by transferring a phosphatidyl group from a second CDP-diacylglycerol to PG. It requires a divalent metal cation cofactor.
|
Metabolite
Metabolic
|
|
|
SMP0449387![]() |
Hexuronide and Hexuronate DegradationMesorhizobium loti MAFF303099
Beta-D-glucuronosides, D-glucuronate and D-fructuronate can be used as a source of carbon for E.coli. They are imported into E.coli's periplasmic space by membrane-associated protein (UidC/gusC), and are further imported into cytoplasm by hydrogen symporter. Beta-glucuronides undergoes hydrolysis by beta-D-glucuronidase to form D-glucuronate. D-glucuronate is isomerized by D-glucuronate isomerase to form D-fructuronate. D-fructuronate is further reduced to D-mannonate by D-mannonate oxidoreductase. D-mannonate dehydratase dehydrated to yield 2-dehydro-3-deoxy-D-gluconate. At this point, a common enzyme, 2-keto-3-deoxygluconokinase, phosphorylates 2-dehydro-3-deoxy-D-gluconate to yield 2-dehydro-3-deoxy-D-gluconate-6-phosphate. This product is then process by KHG/KDPG aldolase which in turn produces D-Glyceraldehyde 3-phosphate and Pyruvic Acid which then go into their respective sub pathways: glycolysis and pyruvate dehydrogenase. The pathway can also start from 3 other points: a hydrogen ion symporter (gluconate/fructuronate transporter GntP) of D-fructuronate, a hydrogen ion symporter (Hexuronate transporter) of aldehydo-D-galacturonate that spontaneously turns into D-tagaturonate. This compound can also be obtained by the reaction of aldehydo-L-galactonate with a NAD dependent l-galactonate oxidoreductase resulting in the release of NADH, hydrogen ion. Tagaturonate then undergoes an NADH-dependent reduction to D-altronate through an altronate oxidoreductase. D-altronate undergoes dehydration to yield 2-dehydro-3-deoxy-D-gluconate, the third and last point where the reaction can start from a hydrogen symporter of a 2-dehydro-3-deoy-D-gluconate.
|
Metabolite
Metabolic
|
|
|
SMP0449380![]() |
beta-Alanine MetabolismLeptotrichia buccalis C-1013-b
Beta-Alanine metabolism starts as a product of aspartate metabolism. Aspartate is decarboxylated by aspartate 1-decarboxylase, releasing carbon dioxide and beta-alanine. Beta-Alanine is then metabolized through a pantothenate synthease resulting in pantothenic acid. Pantothenic acid then undergoes phosphorylation through an ATP-driven pantothenate kinase, resulting in D-4-phosphopantothenate. Pantothenate, vitamin B5, is a precursor for synthesis of 4'-phosphopantetheine moiety of coenzyme A and acyl carrier protein. Plants and microorganisms can synthesize pantothenate de novo, but animals must obtain it from diet. Enzymes of beta-alanine metabolism are targets for anti-microbial drugs.
|
Metabolite
Metabolic
|
||
SMP0449606![]() |
Amino Sugar and Nucleotide Sugar Metabolism IICampylobacter jejuni RM1221
The synthesis of amino sugars and nucleotide sugars starts with the phosphorylation of N-Acetylmuramic acid (MurNac) through its transport from the periplasmic space to the cytoplasm. Once in the cytoplasm, MurNac and water undergo a reversible reaction through a N-acetylmuramic acid 6-phosphate etherase, producing a D-lactic acid and N-Acetyl-D-Glucosamine 6-phosphate. This latter compound can also be introduced into the cytoplasm through a phosphorylating PTS permase in the inner membrane that allows for the transport of N-Acetyl-D-glucosamine from the periplasmic space. N-Acetyl-D-Glucosamine 6-phosphate can also be obtained from chitin dependent reactions. Chitin is hydrated through a bifunctional chitinase to produce chitobiose. This in turn gets hydrated by a beta-hexosaminidase to produce N-acetyl-D-glucosamine. The latter undergoes an atp dependent phosphorylation leading to the production of N-Acetyl-D-Glucosamine 6-phosphate.
N-Acetyl-D-Glucosamine 6-phosphate is then be deacetylated in order to produce Glucosamine 6-phosphate through a N-acetylglucosamine-6-phosphate deacetylase. This compound can either be isomerized or deaminated into Beta-D-fructofuranose 6-phosphate through a glucosamine-fructose-6-phosphate aminotransferase and a glucosamine-6-phosphate deaminase respectively.
Glucosamine 6-phosphate undergoes a reversible reaction to glucosamine 1 phosphate through a phosphoglucosamine mutase. This compound is then acetylated through a bifunctional protein glmU to produce a N-Acetyl glucosamine 1-phosphate.
N-Acetyl glucosamine 1-phosphate enters the nucleotide sugar synthesis by reacting with UTP and hydrogen ion through a bifunctional protein glmU releasing pyrophosphate and a Uridine diphosphate-N-acetylglucosamine.This compound can either be isomerized into a UDP-N-acetyl-D-mannosamine or undergo a reaction with phosphoenolpyruvic acid through UDP-N-acetylglucosamine 1-carboxyvinyltransferase releasing a phosphate and a UDP-N-Acetyl-alpha-D-glucosamine-enolpyruvate.
UDP-N-acetyl-D-mannosamine undergoes a NAD dependent dehydrogenation through a UDP-N-acetyl-D-mannosamine dehydrogenase, releasing NADH, a hydrogen ion and a UDP-N-Acetyl-alpha-D-mannosaminuronate, This compound is then used in the production of enterobacterial common antigens.
UDP-N-Acetyl-alpha-D-glucosamine-enolpyruvate is reduced through a NADPH dependent UDP-N-acetylenolpyruvoylglucosamine reductase, releasing a NADP and a UDP-N-acetyl-alpha-D-muramate. This compound is involved in the D-glutamine and D-glutamate metabolism.
|
Metabolite
Metabolic
|
|
Showing 371441 -
371450 of 371729 pathways