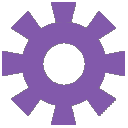
Browsing Pathways
Showing 371551 -
371560 of 605359 pathways
PathBank ID | Pathway Name and Description | Pathway Class | Chemical Compounds | Proteins |
---|---|---|---|---|
SMP0342723 |
Cardiolipin Biosynthesis CL(18:2(9Z,12Z)/22:6(4Z,7Z,10Z,13Z,16Z,19Z)/16:1(9Z)/20:2(11Z,14Z))Mus musculus
Cardiolipin (CL) is an important component of the inner mitochondrial membrane where it constitutes about 20% of the total lipid composition. It is essential for the optimal function of numerous enzymes that are involved in mitochondrial energy metabolism (Wikipedia). Cardiolipin biosynthesis occurs mainly in the mitochondria, but there also exists an alternative synthesis route for CDP-diacylglycerol that takes place in the endoplasmic reticulum. This second route may supplement this pathway. All membrane-localized enzymes are coloured dark green in the image. First, dihydroxyacetone phosphate (or glycerone phosphate) from glycolysis is used by the cytosolic enzyme glycerol-3-phosphate dehydrogenase [NAD(+)] to synthesize sn-glycerol 3-phosphate. Second, the mitochondrial outer membrane enzyme glycerol-3-phosphate acyltransferase esterifies an acyl-group to the sn-1 position of sn-glycerol 3-phosphate to form 1-acyl-sn-glycerol 3-phosphate (lysophosphatidic acid or LPA). Third, the enzyme 1-acyl-sn-glycerol-3-phosphate acyltransferase converts LPA into phosphatidic acid (PA or 1,2-diacyl-sn-glycerol 3-phosphate) by esterifying an acyl-group to the sn-2 position of the glycerol backbone. PA is then transferred to the inner mitochondrial membrane to continue cardiolipin synthesis. Fourth, magnesium-dependent phosphatidate cytidylyltransferase catalyzes the conversion of PA into CDP-diacylglycerol. Fifth, CDP-diacylglycerol--glycerol-3-phosphate 3-phosphatidyltransferase synthesizes phosphatidylglycerophosphate (PGP). Sixth, phosphatidylglycerophosphatase and protein-tyrosine phosphatase dephosphorylates PGP to form phosphatidylglycerol (PG). Last, cardiolipin synthase catalyzes the synthesis of cardiolipin by transferring a phosphatidyl group from a second CDP-diacylglycerol to PG. It requires a divalent metal cation cofactor.
|
Metabolite
Metabolic
|
|
|
SMP0342716 |
Cardiolipin Biosynthesis CL(18:2(9Z,12Z)/22:6(4Z,7Z,10Z,13Z,16Z,19Z)/16:1(9Z)/18:0)Mus musculus
Cardiolipin (CL) is an important component of the inner mitochondrial membrane where it constitutes about 20% of the total lipid composition. It is essential for the optimal function of numerous enzymes that are involved in mitochondrial energy metabolism (Wikipedia). Cardiolipin biosynthesis occurs mainly in the mitochondria, but there also exists an alternative synthesis route for CDP-diacylglycerol that takes place in the endoplasmic reticulum. This second route may supplement this pathway. All membrane-localized enzymes are coloured dark green in the image. First, dihydroxyacetone phosphate (or glycerone phosphate) from glycolysis is used by the cytosolic enzyme glycerol-3-phosphate dehydrogenase [NAD(+)] to synthesize sn-glycerol 3-phosphate. Second, the mitochondrial outer membrane enzyme glycerol-3-phosphate acyltransferase esterifies an acyl-group to the sn-1 position of sn-glycerol 3-phosphate to form 1-acyl-sn-glycerol 3-phosphate (lysophosphatidic acid or LPA). Third, the enzyme 1-acyl-sn-glycerol-3-phosphate acyltransferase converts LPA into phosphatidic acid (PA or 1,2-diacyl-sn-glycerol 3-phosphate) by esterifying an acyl-group to the sn-2 position of the glycerol backbone. PA is then transferred to the inner mitochondrial membrane to continue cardiolipin synthesis. Fourth, magnesium-dependent phosphatidate cytidylyltransferase catalyzes the conversion of PA into CDP-diacylglycerol. Fifth, CDP-diacylglycerol--glycerol-3-phosphate 3-phosphatidyltransferase synthesizes phosphatidylglycerophosphate (PGP). Sixth, phosphatidylglycerophosphatase and protein-tyrosine phosphatase dephosphorylates PGP to form phosphatidylglycerol (PG). Last, cardiolipin synthase catalyzes the synthesis of cardiolipin by transferring a phosphatidyl group from a second CDP-diacylglycerol to PG. It requires a divalent metal cation cofactor.
|
Metabolite
Metabolic
|
|
|
SMP0342721 |
Cardiolipin Biosynthesis CL(18:2(9Z,12Z)/22:6(4Z,7Z,10Z,13Z,16Z,19Z)/16:1(9Z)/18:4(6Z,9Z,12Z,15Z))Mus musculus
Cardiolipin (CL) is an important component of the inner mitochondrial membrane where it constitutes about 20% of the total lipid composition. It is essential for the optimal function of numerous enzymes that are involved in mitochondrial energy metabolism (Wikipedia). Cardiolipin biosynthesis occurs mainly in the mitochondria, but there also exists an alternative synthesis route for CDP-diacylglycerol that takes place in the endoplasmic reticulum. This second route may supplement this pathway. All membrane-localized enzymes are coloured dark green in the image. First, dihydroxyacetone phosphate (or glycerone phosphate) from glycolysis is used by the cytosolic enzyme glycerol-3-phosphate dehydrogenase [NAD(+)] to synthesize sn-glycerol 3-phosphate. Second, the mitochondrial outer membrane enzyme glycerol-3-phosphate acyltransferase esterifies an acyl-group to the sn-1 position of sn-glycerol 3-phosphate to form 1-acyl-sn-glycerol 3-phosphate (lysophosphatidic acid or LPA). Third, the enzyme 1-acyl-sn-glycerol-3-phosphate acyltransferase converts LPA into phosphatidic acid (PA or 1,2-diacyl-sn-glycerol 3-phosphate) by esterifying an acyl-group to the sn-2 position of the glycerol backbone. PA is then transferred to the inner mitochondrial membrane to continue cardiolipin synthesis. Fourth, magnesium-dependent phosphatidate cytidylyltransferase catalyzes the conversion of PA into CDP-diacylglycerol. Fifth, CDP-diacylglycerol--glycerol-3-phosphate 3-phosphatidyltransferase synthesizes phosphatidylglycerophosphate (PGP). Sixth, phosphatidylglycerophosphatase and protein-tyrosine phosphatase dephosphorylates PGP to form phosphatidylglycerol (PG). Last, cardiolipin synthase catalyzes the synthesis of cardiolipin by transferring a phosphatidyl group from a second CDP-diacylglycerol to PG. It requires a divalent metal cation cofactor.
|
Metabolite
Metabolic
|
|
|
SMP0447904![]() |
Secondary Metabolites: Glyoxylate CycleAlcaligenes faecalis subsp. faecalis NCIB 8687
The glyoxylate cycle starts with the interaction of Acetyl-Coa with a water molecule and Oxalacetic acid interact through a Citrate synthase resulting in a release of a coenzyme a and citric acid. The citric acid gets dehydrated through a citrate hydro-lyase resulting in the release of a water molecule and cis-Aconitic acid. The cis-Aconitic acid is then hydrated in an reversible reaction through an aconitate hydratase resulting in an Isocitric acid. The isocitric acid then interacts in a reversible reaction through isocitrate lyase resulting in the release of a succinic acid and a glyoxylic acid. The glyoxylic acid then reacts in a reversible reaction with an acetyl-coa, and a water molecule in a reversible reaction, resulting in a release of a coenzyme A, a hydrogen ion and an L-malic acid. The L-malic acid interacts in a reversible reaction through a NAD driven malate dehydrogenase resulting in the release of NADH, a hydrogen ion and an Oxalacetic acid.
|
Metabolite
Metabolic
|
||
SMP0447910![]() |
Secondary Metabolites: Trehalose Biosynthesis and MetabolismBordetella hinzii OH87 BAL007II
Threhalose biosynthesis begins with an Alpha-D-glucose-1-phosphate interacting with an ATP through a glucose-1-phosphate adenylyltransferase resulting in the release of a pyrophosphate and an ADP-glucose. The latter compound interacts in a reversible reaction with an amylose through a glycogen synthase resulting in the release of an ADP and an amylose. Amylose then interacts in a reversible reaction with 1,4-α-glucan branching enzyme resulting in a glycogen
Glycogen can also be produced by a reversible reaction with Amylose through a maltodextrin phosphorylase, releasing a phosphate and a glycogen.
Glycogen is then transformed into trehalose through a glycogen debranching enzyme. Alpha Alpha Trehalose can be degraded by reacting with with a water molecule through a cytoplasmic trehalase resulting in the release of a Beta-D-glucose and an Alpha-D-glucose.phosphorylated resulting in a Beta-D-glucose 6-phosphate. This compound is phosphorylated and can then join glycolysis
Alpha Alpha Trehalose can be degraded in the periplasmic space by reacting with with a water molecule through a periplasmic trehalase resulting in the release of a Beta-D-glucose and an Alpha-D-glucose.
The beta-D-glucose can be transported into the cytosol through a PTS permease where it is phosphorylated resulting in a Beta-D-glucose 6-phosphate. This compound can then join glycolysis
|
Metabolite
Metabolic
|
||
SMP0447906![]() |
Hexuronide and Hexuronate DegradationBacteroides sp. 2_1_33B
Beta-D-glucuronosides, D-glucuronate and D-fructuronate can be used as a source of carbon for E.coli. They are imported into E.coli's periplasmic space by membrane-associated protein (UidC/gusC), and are further imported into cytoplasm by hydrogen symporter. Beta-glucuronides undergoes hydrolysis by beta-D-glucuronidase to form D-glucuronate. D-glucuronate is isomerized by D-glucuronate isomerase to form D-fructuronate. D-fructuronate is further reduced to D-mannonate by D-mannonate oxidoreductase. D-mannonate dehydratase dehydrated to yield 2-dehydro-3-deoxy-D-gluconate. At this point, a common enzyme, 2-keto-3-deoxygluconokinase, phosphorylates 2-dehydro-3-deoxy-D-gluconate to yield 2-dehydro-3-deoxy-D-gluconate-6-phosphate. This product is then process by KHG/KDPG aldolase which in turn produces D-Glyceraldehyde 3-phosphate and Pyruvic Acid which then go into their respective sub pathways: glycolysis and pyruvate dehydrogenase. The pathway can also start from 3 other points: a hydrogen ion symporter (gluconate/fructuronate transporter GntP) of D-fructuronate, a hydrogen ion symporter (Hexuronate transporter) of aldehydo-D-galacturonate that spontaneously turns into D-tagaturonate. This compound can also be obtained by the reaction of aldehydo-L-galactonate with a NAD dependent l-galactonate oxidoreductase resulting in the release of NADH, hydrogen ion. Tagaturonate then undergoes an NADH-dependent reduction to D-altronate through an altronate oxidoreductase. D-altronate undergoes dehydration to yield 2-dehydro-3-deoxy-D-gluconate, the third and last point where the reaction can start from a hydrogen symporter of a 2-dehydro-3-deoy-D-gluconate.
|
Metabolite
Metabolic
|
|
|
SMP0448152![]() |
Propanoate MetabolismNeisseria elongata subsp. glycolytica ATCC 29315
Starting from L-threonine, this compound is deaminated through a threonine deaminase resulting in a hydrogen ion, a water molecule and a (2z)-2-aminobut-2-enoate. The latter compound then isomerizes to a 2-iminobutanoate, This compound then reacts spontaneously with hydrogen ion and a water molecule resulting in a ammonium and a 2-Ketobutyric acid. The latter compound interacts with CoA through a pyruvate formate-lyase / 2-ketobutyrate formate-lyase resulting in a formic acid and a propionyl-CoA.
Propionyl-CoA can then be processed either into a 2-methylcitric acid or into a propanoyl phosphate. Propionyl-CoA interacts with oxalacetic acid and a water molecule through a 2-methylcitrate synthase resulting in a hydrogen ion, a CoA and a 2-Methylcitric acid.The latter compound is dehydrated through a 2-methylcitrate dehydratase resulting in a water molecule and cis-2-methylaconitate. The latter compound is then dehydrated by a bifunctional aconitate hydratase 2 and 2-methylisocitrate dehydratase resulting in a water molecule and methylisocitric acid. The latter compound is then processed by 2-methylisocitrate lyase resulting in a release of succinic acid and pyruvic acid. Succinic acid can then interact with a propionyl-CoA through a propionyl-CoA:succinate CoA transferase resulting in a propionic acid and a succinyl CoA. Succinyl-CoA is then isomerized through a methylmalonyl-CoA mutase resulting in a methylmalonyl-CoA. This compound is then decarboxylated through a methylmalonyl-CoA decarboxylase resulting in a release of Carbon dioxide and Propionyl-CoA. Propionyl-CoA interacts with a phosphate through a phosphate acetyltransferase / phosphate propionyltransferase resulting in a CoA and a propanoyl phosphate. Propionyl-CoA can react with a phosphate through a phosphate acetyltransferase / phosphate propionyltransferase resulting in a CoA and a propanoyl phosphate. The latter compound is then dephosphorylated through a ADP driven acetate kinase/propionate kinase protein complex resulting in an ATP and Propionic acid. Propionic acid can be processed by a reaction with CoA through a ATP-driven propionyl-CoA synthetase resulting in a pyrophosphate, an AMP and a propionyl-CoA.
|
Metabolite
Metabolic
|
|
|
SMP0448152![]() |
Propanoate MetabolismNeisseria elongata subsp. glycolytica ATCC 29315
Starting from L-threonine, this compound is deaminated through a threonine deaminase resulting in a hydrogen ion, a water molecule and a (2z)-2-aminobut-2-enoate. The latter compound then isomerizes to a 2-iminobutanoate, This compound then reacts spontaneously with hydrogen ion and a water molecule resulting in a ammonium and a 2-Ketobutyric acid. The latter compound interacts with CoA through a pyruvate formate-lyase / 2-ketobutyrate formate-lyase resulting in a formic acid and a propionyl-CoA.
Propionyl-CoA can then be processed either into a 2-methylcitric acid or into a propanoyl phosphate. Propionyl-CoA interacts with oxalacetic acid and a water molecule through a 2-methylcitrate synthase resulting in a hydrogen ion, a CoA and a 2-Methylcitric acid.The latter compound is dehydrated through a 2-methylcitrate dehydratase resulting in a water molecule and cis-2-methylaconitate. The latter compound is then dehydrated by a bifunctional aconitate hydratase 2 and 2-methylisocitrate dehydratase resulting in a water molecule and methylisocitric acid. The latter compound is then processed by 2-methylisocitrate lyase resulting in a release of succinic acid and pyruvic acid. Succinic acid can then interact with a propionyl-CoA through a propionyl-CoA:succinate CoA transferase resulting in a propionic acid and a succinyl CoA. Succinyl-CoA is then isomerized through a methylmalonyl-CoA mutase resulting in a methylmalonyl-CoA. This compound is then decarboxylated through a methylmalonyl-CoA decarboxylase resulting in a release of Carbon dioxide and Propionyl-CoA. Propionyl-CoA interacts with a phosphate through a phosphate acetyltransferase / phosphate propionyltransferase resulting in a CoA and a propanoyl phosphate. Propionyl-CoA can react with a phosphate through a phosphate acetyltransferase / phosphate propionyltransferase resulting in a CoA and a propanoyl phosphate. The latter compound is then dephosphorylated through a ADP driven acetate kinase/propionate kinase protein complex resulting in an ATP and Propionic acid. Propionic acid can be processed by a reaction with CoA through a ATP-driven propionyl-CoA synthetase resulting in a pyrophosphate, an AMP and a propionyl-CoA.
|
Metabolite
Metabolic
|
|
|
SMP0448161![]() |
Pyrimidine MetabolismNeisseria flavescens SK114
The metabolism of pyrimidines begins with L-glutamine interacting with water molecule and a hydrogen carbonate through an ATP driven carbamoyl phosphate synthetase resulting in a hydrogen ion, an ADP, a phosphate, an L-glutamic acid and a carbamoyl phosphate. The latter compound interacts with an L-aspartic acid through a aspartate transcarbamylase resulting in a phosphate, a hydrogen ion and a N-carbamoyl-L-aspartate. The latter compound interacts with a hydrogen ion through a dihydroorotase resulting in the release of a water molecule and a 4,5-dihydroorotic acid. This compound interacts with an ubiquinone-1 through a dihydroorotate dehydrogenase, type 2 resulting in a release of an ubiquinol-1 and an orotic acid. The orotic acid then interacts with a phosphoribosyl pyrophosphate through a orotate phosphoribosyltransferase resulting in a pyrophosphate and an orotidylic acid. The latter compound then interacts with a hydrogen ion through an orotidine-5 '-phosphate decarboxylase, resulting in an release of carbon dioxide and an Uridine 5' monophosphate. The Uridine 5' monophosphate process to get phosphorylated by an ATP driven UMP kinase resulting in the release of an ADP and an Uridine 5--diphosphate. Uridine 5-diphosphate can be metabolized in multiple ways in order to produce a Deoxyuridine triphosphate. 1.-Uridine 5-diphosphate interacts with a reduced thioredoxin through a ribonucleoside diphosphate reductase 1 resulting in the release of a water molecule and an oxidized thioredoxin and an dUDP. The dUDP is then phosphorylated by an ATP through a nucleoside diphosphate kinase resulting in the release of an ADP and a DeoxyUridine triphosphate. 2.-Uridine 5-diphosphate interacts with a reduced NrdH glutaredoxin-like protein through a Ribonucleoside-diphosphate reductase 1 resulting in a release of a water molecule, an oxidized NrdH glutaredoxin-like protein and a dUDP. The dUDP is then phosphorylated by an ATP through a nucleoside diphosphate kinase resulting in the release of an ADP and a DeoxyUridine triphosphate. 3.-Uridine 5-diphosphate is phosphorylated by an ATP-driven nucleoside diphosphate kinase resulting in an ADP and an Uridinetriphosphate. The latter compound interacts with a reduced flavodoxin through ribonucleoside-triphosphate reductase resulting in the release of an oxidized flavodoxin, a water molecule and a Deoxyuridine triphosphate 4.-Uridine 5-diphosphate is phosphorylated by an ATP-driven nucleoside diphosphate kinase resulting in an ADP and an Uridinetriphosphate The uridine triphosphate interacts with a L-glutamine and a water molecule through an ATP driven CTP synthase resulting in an ADP, a phosphate, a hydrogen ion, an L-glutamic acid and a cytidine triphosphate. The cytidine triphosphate interacts with a reduced flavodoxin through a ribonucleoside-triphosphate reductase resulting in the release of a water molecule, an oxidized flavodoxin and a dCTP. The dCTP interacts with a water molecule and a hydrogen ion through a dCTP deaminase resulting in a release of an ammonium molecule and a Deoxyuridine triphosphate. 5.-Uridine 5-diphosphate is phosphorylated by an ATP-driven nucleoside diphosphate kinase resulting in an ADP and an Uridinetriphosphate The uridine triphosphate interacts with a L-glutamine and a water molecule through an ATP driven CTP synthase resulting in an ADP, a phosphate, a hydrogen ion, an L-glutamic acid and a cytidine triphosphate. The cytidine triphosphate then interacts spontaneously with a water molecule resulting in the release of a phosphate, a hydrogen ion and a CDP. The CDP then interacts with a reduced NrdH glutaredoxin-like protein through a ribonucleoside-diphosphate reductase 2 resulting in the release of a water molecule, an oxidized NrdH glutaredoxin-like protein and a dCDP. The dCDP is then phosphorylated through an ATP driven nucleoside diphosphate kinase resulting in an ADP and a dCTP. The dCTP interacts with a water molecule and a hydrogen ion through a dCTP deaminase resulting in a release of an ammonium molecule and a Deoxyuridine triphosphate. 6.-Uridine 5-diphosphate is phosphorylated by an ATP-driven nucleoside diphosphate kinase resulting in an ADP and an Uridinetriphosphate The uridine triphosphate interacts with a L-glutamine and a water molecule through an ATP driven CTP synthase resulting in an ADP, a phosphate, a hydrogen ion, an L-glutamic acid and a cytidine triphosphate. The cytidine triphosphate then interacts spontaneously with a water molecule resulting in the release of a phosphate, a hydrogen ion and a CDP. The CDP interacts with a reduced thioredoxin through a ribonucleoside diphosphate reductase 1 resulting in a release of a water molecule, an oxidized thioredoxin and a dCDP. The dCDP is then phosphorylated through an ATP driven nucleoside diphosphate kinase resulting in an ADP and a dCTP. The dCTP interacts with a water molecule and a hydrogen ion through a dCTP deaminase resulting in a release of an ammonium molecule and a Deoxyuridine triphosphate. The deoxyuridine triphosphate then interacts with a water molecule through a nucleoside triphosphate pyrophosphohydrolase resulting in a release of a hydrogen ion, a phosphate and a dUMP. The dUMP then interacts with a methenyltetrahydrofolate through a thymidylate synthase resulting in a dihydrofolic acid and a 5-thymidylic acid. Then 5-thymidylic acid is then phosphorylated through a nucleoside diphosphate kinase resulting in the release of an ADP and thymidine 5'-triphosphate.
|
Metabolite
Metabolic
|
|
|
SMP0342969 |
Cardiolipin Biosynthesis CL(18:4(6Z,9Z,12Z,15Z)/22:6(4Z,7Z,10Z,13Z,16Z,19Z)/16:1(9Z)/22:6(4Z,7Z,10Z,13Z,16Z,19Z))Mus musculus
Cardiolipin (CL) is an important component of the inner mitochondrial membrane where it constitutes about 20% of the total lipid composition. It is essential for the optimal function of numerous enzymes that are involved in mitochondrial energy metabolism (Wikipedia). Cardiolipin biosynthesis occurs mainly in the mitochondria, but there also exists an alternative synthesis route for CDP-diacylglycerol that takes place in the endoplasmic reticulum. This second route may supplement this pathway. All membrane-localized enzymes are coloured dark green in the image. First, dihydroxyacetone phosphate (or glycerone phosphate) from glycolysis is used by the cytosolic enzyme glycerol-3-phosphate dehydrogenase [NAD(+)] to synthesize sn-glycerol 3-phosphate. Second, the mitochondrial outer membrane enzyme glycerol-3-phosphate acyltransferase esterifies an acyl-group to the sn-1 position of sn-glycerol 3-phosphate to form 1-acyl-sn-glycerol 3-phosphate (lysophosphatidic acid or LPA). Third, the enzyme 1-acyl-sn-glycerol-3-phosphate acyltransferase converts LPA into phosphatidic acid (PA or 1,2-diacyl-sn-glycerol 3-phosphate) by esterifying an acyl-group to the sn-2 position of the glycerol backbone. PA is then transferred to the inner mitochondrial membrane to continue cardiolipin synthesis. Fourth, magnesium-dependent phosphatidate cytidylyltransferase catalyzes the conversion of PA into CDP-diacylglycerol. Fifth, CDP-diacylglycerol--glycerol-3-phosphate 3-phosphatidyltransferase synthesizes phosphatidylglycerophosphate (PGP). Sixth, phosphatidylglycerophosphatase and protein-tyrosine phosphatase dephosphorylates PGP to form phosphatidylglycerol (PG). Last, cardiolipin synthase catalyzes the synthesis of cardiolipin by transferring a phosphatidyl group from a second CDP-diacylglycerol to PG. It requires a divalent metal cation cofactor.
|
Metabolite
Metabolic
|
|
Showing 371551 -
371560 of 372176 pathways