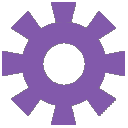
Browsing Pathways
Showing 371721 -
371730 of 605359 pathways
PathBank ID | Pathway Name and Description | Pathway Class | Chemical Compounds | Proteins |
---|---|---|---|---|
SMP0448871![]() |
Colanic Acid Building Blocks BiosynthesisEnterobacter cancerogenus ATCC 35316
The colonic acid building blocks biosynthesis starts with a Beta-D-Glucose undergoing a transport reaction mediated by a glucose PTS permease. The permease phosphorylates the Beta-D-Glucose, producing a Beta-D-Glucose 6-phosphate. This compound can either change to an Alpha-D-Glucose 6-phosphate spontaneously or into a fructose 6-phosphate through a glucose-6-phosphate isomerase. The latter compound can also be present in E.coli through the interaction of D-fructose and a mannose PTS permease which phosphorylate the D-fructose.
Fructose 6-phosphate interacts in a reversible reaction with mannose-6-phosphate isomerase in order to produce a Alpha-D-mannose 6-phosphate. This compound can also be present in E.coli through the interaction of Alpha-D-mannose and a mannose PTS permease which phosphorylates the alpha-D-mannose. Alpha-D-mannose 6-phosphate interacts in a reversible reaction with a phosphomannomutase to produce a alpha-D-mannose 1-phosphate. This compound in turn with a hydrogen ion and gtp undergoes a reaction with a mannose-1-phosphate guanylyltransferase, releasing a pyrophosphate and producing a guanosine diphosphate mannose. Guanosine diphosphate mannose interacts with gdp-mannose 4,6-dehydratase releasing a water, and gdp-4-dehydro-6-deoxy-D-mannose. This compound in turn with hydrogen ion and NADPH interact with GDP-L-fucose synthase releasing NADP and producing a GDP-L-fucose.
The Alpha-D-Glucose 6-phosphate interacts in a reversible reaction with phosphoglucomutase-1 to produce a alpha-D-glucose 1-phosphate. This in turn with UTP and hydrogen ion interact with UTP--glucose-1-phosphate uridyleltransferase releasing a pyrophosphate and UDP-glucose.
UDP-glucose can either interact with galactose-1-phosphate uridylyltransferase to produce a UDP-galactose or in turn with NAD and water interact with UDP-glucose 6-dehydrogenase releasing a NADH and a hydrogen ion and producing a UDP-glucuronate.
GDP-L-fucose, UDP-glucose, UDP-galactose and UDP-glucuronate are sugars that need to be activated in the form of nucleotide sugar prior to their assembly into colanic acid, also known as M antigen.
Colanic acid is an extracellular polysaccharide which has been linked to a cluster of 19 genes(wca).
|
Metabolite
Metabolic
|
|
|
SMP0448888![]() |
Secondary Metabolites: Enterobacterial Common Antigen BiosynthesisCitrobacter youngae ATCC 29220
The biosynthesis of a enterobacterial common antigen can begin with a di-trans,octa-cis-undecaprenyl phosphate interacts with a Uridine diphosphate-N-acetylglucosamine through undecaprenyl-phosphate α-N-acetylglucosaminyl transferase resulting in a N-acetyl-α-D-glucosaminyl-diphospho-ditrans,octacis-undecaprenol and a Uridine 5'-monophosphate. The N-acetyl-α-D-glucosaminyl-diphospho-ditrans,octacis-undecaprenol then reacts with an UDP-ManNAcA from the Amino sugar and nucleotide sugar metabolism pathway. This reaction is metabolized by a UDP-N-acetyl-D-mannosaminuronic acid transferase resulting in a uridine 5' diphosphate, a hydrogen ion and a Undecaprenyl N-acetyl-glucosaminyl-N-acetyl-mannosaminuronate-4-acetamido-4,6-dideoxy-D-galactose pyrophosphate. Glucose 1 phosphate can be metabolize by interacting with a hydrogen ion and a thymidine 5-triphosphate by either reacting with a dTDP-glucose pyrophosphorylase or a dTDP-glucose pyrophosphorylase 2 resulting in the release of a pyrophosphate and a dTDP-D-glucose. The latter compound is then dehydrated through an dTDP-glucose 4,6-dehydratase 2 resulting in water and dTDP-4-dehydro-6-deoxy-D-glucose. The latter compound interacts with L-glutamic acid through a dTDP-4-dehydro-6-deoxy-D-glucose transaminase resulting in the release of oxoglutaric acid and dTDP-thomosamine. The latter compound interacts with acetyl-coa through a dTDP-fucosamine acetyltransferase resulting in a Coenzyme A, a hydrogen Ion and a TDP-Fuc4NAc. Undecaprenyl N-acetyl-glucosaminyl-N-acetyl-mannosaminuronate-4-acetamido-4,6-dideoxy-D-galactose pyrophosphate then interacts with a TDP--Fuc4NAc through a 4-acetamido-4,6-dideoxy-D-galactose transferase resulting in a hydrogen ion, a dTDP and a Undecaprenyl N-acetyl-glucosaminyl-N-acetyl-mannosaminuronate-4-acetamido-4,6-dideoxy-D-galactose pyrophosphate. This compound is then transported through a protein wzxE into the periplasmic space so that it can be incorporated into the outer membrane.
|
Metabolite
Metabolic
|
|
|
SMP0343568 |
Cardiolipin Biosynthesis CL(20:3(11Z,14Z,17Z)/22:6(4Z,7Z,10Z,13Z,16Z,19Z)/20:2(11Z,14Z)/20:4(5Z,8Z,11Z,14Z))Mus musculus
Cardiolipin (CL) is an important component of the inner mitochondrial membrane where it constitutes about 20% of the total lipid composition. It is essential for the optimal function of numerous enzymes that are involved in mitochondrial energy metabolism (Wikipedia). Cardiolipin biosynthesis occurs mainly in the mitochondria, but there also exists an alternative synthesis route for CDP-diacylglycerol that takes place in the endoplasmic reticulum. This second route may supplement this pathway. All membrane-localized enzymes are coloured dark green in the image. First, dihydroxyacetone phosphate (or glycerone phosphate) from glycolysis is used by the cytosolic enzyme glycerol-3-phosphate dehydrogenase [NAD(+)] to synthesize sn-glycerol 3-phosphate. Second, the mitochondrial outer membrane enzyme glycerol-3-phosphate acyltransferase esterifies an acyl-group to the sn-1 position of sn-glycerol 3-phosphate to form 1-acyl-sn-glycerol 3-phosphate (lysophosphatidic acid or LPA). Third, the enzyme 1-acyl-sn-glycerol-3-phosphate acyltransferase converts LPA into phosphatidic acid (PA or 1,2-diacyl-sn-glycerol 3-phosphate) by esterifying an acyl-group to the sn-2 position of the glycerol backbone. PA is then transferred to the inner mitochondrial membrane to continue cardiolipin synthesis. Fourth, magnesium-dependent phosphatidate cytidylyltransferase catalyzes the conversion of PA into CDP-diacylglycerol. Fifth, CDP-diacylglycerol--glycerol-3-phosphate 3-phosphatidyltransferase synthesizes phosphatidylglycerophosphate (PGP). Sixth, phosphatidylglycerophosphatase and protein-tyrosine phosphatase dephosphorylates PGP to form phosphatidylglycerol (PG). Last, cardiolipin synthase catalyzes the synthesis of cardiolipin by transferring a phosphatidyl group from a second CDP-diacylglycerol to PG. It requires a divalent metal cation cofactor.
|
Metabolite
Metabolic
|
|
|
SMP0343575 |
Cardiolipin Biosynthesis CL(20:3(11Z,14Z,17Z)/22:6(4Z,7Z,10Z,13Z,16Z,19Z)/20:3(11Z,14Z,17Z)/16:1(9Z))Mus musculus
Cardiolipin (CL) is an important component of the inner mitochondrial membrane where it constitutes about 20% of the total lipid composition. It is essential for the optimal function of numerous enzymes that are involved in mitochondrial energy metabolism (Wikipedia). Cardiolipin biosynthesis occurs mainly in the mitochondria, but there also exists an alternative synthesis route for CDP-diacylglycerol that takes place in the endoplasmic reticulum. This second route may supplement this pathway. All membrane-localized enzymes are coloured dark green in the image. First, dihydroxyacetone phosphate (or glycerone phosphate) from glycolysis is used by the cytosolic enzyme glycerol-3-phosphate dehydrogenase [NAD(+)] to synthesize sn-glycerol 3-phosphate. Second, the mitochondrial outer membrane enzyme glycerol-3-phosphate acyltransferase esterifies an acyl-group to the sn-1 position of sn-glycerol 3-phosphate to form 1-acyl-sn-glycerol 3-phosphate (lysophosphatidic acid or LPA). Third, the enzyme 1-acyl-sn-glycerol-3-phosphate acyltransferase converts LPA into phosphatidic acid (PA or 1,2-diacyl-sn-glycerol 3-phosphate) by esterifying an acyl-group to the sn-2 position of the glycerol backbone. PA is then transferred to the inner mitochondrial membrane to continue cardiolipin synthesis. Fourth, magnesium-dependent phosphatidate cytidylyltransferase catalyzes the conversion of PA into CDP-diacylglycerol. Fifth, CDP-diacylglycerol--glycerol-3-phosphate 3-phosphatidyltransferase synthesizes phosphatidylglycerophosphate (PGP). Sixth, phosphatidylglycerophosphatase and protein-tyrosine phosphatase dephosphorylates PGP to form phosphatidylglycerol (PG). Last, cardiolipin synthase catalyzes the synthesis of cardiolipin by transferring a phosphatidyl group from a second CDP-diacylglycerol to PG. It requires a divalent metal cation cofactor.
|
Metabolite
Metabolic
|
|
|
SMP0343580 |
Cardiolipin Biosynthesis CL(20:3(11Z,14Z,17Z)/22:6(4Z,7Z,10Z,13Z,16Z,19Z)/20:3(11Z,14Z,17Z)/20:2(11Z,14Z))Mus musculus
Cardiolipin (CL) is an important component of the inner mitochondrial membrane where it constitutes about 20% of the total lipid composition. It is essential for the optimal function of numerous enzymes that are involved in mitochondrial energy metabolism (Wikipedia). Cardiolipin biosynthesis occurs mainly in the mitochondria, but there also exists an alternative synthesis route for CDP-diacylglycerol that takes place in the endoplasmic reticulum. This second route may supplement this pathway. All membrane-localized enzymes are coloured dark green in the image. First, dihydroxyacetone phosphate (or glycerone phosphate) from glycolysis is used by the cytosolic enzyme glycerol-3-phosphate dehydrogenase [NAD(+)] to synthesize sn-glycerol 3-phosphate. Second, the mitochondrial outer membrane enzyme glycerol-3-phosphate acyltransferase esterifies an acyl-group to the sn-1 position of sn-glycerol 3-phosphate to form 1-acyl-sn-glycerol 3-phosphate (lysophosphatidic acid or LPA). Third, the enzyme 1-acyl-sn-glycerol-3-phosphate acyltransferase converts LPA into phosphatidic acid (PA or 1,2-diacyl-sn-glycerol 3-phosphate) by esterifying an acyl-group to the sn-2 position of the glycerol backbone. PA is then transferred to the inner mitochondrial membrane to continue cardiolipin synthesis. Fourth, magnesium-dependent phosphatidate cytidylyltransferase catalyzes the conversion of PA into CDP-diacylglycerol. Fifth, CDP-diacylglycerol--glycerol-3-phosphate 3-phosphatidyltransferase synthesizes phosphatidylglycerophosphate (PGP). Sixth, phosphatidylglycerophosphatase and protein-tyrosine phosphatase dephosphorylates PGP to form phosphatidylglycerol (PG). Last, cardiolipin synthase catalyzes the synthesis of cardiolipin by transferring a phosphatidyl group from a second CDP-diacylglycerol to PG. It requires a divalent metal cation cofactor.
|
Metabolite
Metabolic
|
|
|
SMP0436456![]() |
Amino Sugar and Nucleotide Sugar Metabolism IEscherichia coli IAI39
The synthesis of amino sugars and nucleotide sugars starts with the phosphorylation of N-Acetylmuramic acid (MurNac) through its transport from the periplasmic space to the cytoplasm. Once in the cytoplasm, MurNac and water undergo a reversible reaction through a N-acetylmuramic acid 6-phosphate etherase, producing a D-lactic acid and N-Acetyl-D-Glucosamine 6-phosphate. This latter compound can also be introduced into the cytoplasm through a phosphorylating PTS permase in the inner membrane that allows for the transport of N-Acetyl-D-glucosamine from the periplasmic space. N-Acetyl-D-Glucosamine 6-phosphate can also be obtained from chitin dependent reactions. Chitin is hydrated through a bifunctional chitinase to produce chitobiose. This in turn gets hydrated by a beta-hexosaminidase to produce N-acetyl-D-glucosamine. The latter undergoes an atp dependent phosphorylation leading to the production of N-Acetyl-D-Glucosamine 6-phosphate.
N-Acetyl-D-Glucosamine 6-phosphate is then be deacetylated in order to produce Glucosamine 6-phosphate through a N-acetylglucosamine-6-phosphate deacetylase. This compound can either be isomerized or deaminated into Beta-D-fructofuranose 6-phosphate through a glucosamine-fructose-6-phosphate aminotransferase and a glucosamine-6-phosphate deaminase respectively.
Glucosamine 6-phosphate undergoes a reversible reaction to glucosamine 1 phosphate through a phosphoglucosamine mutase. This compound is then acetylated through a bifunctional protein glmU to produce a N-Acetyl glucosamine 1-phosphate.
N-Acetyl glucosamine 1-phosphate enters the nucleotide sugar synthesis by reacting with UTP and hydrogen ion through a bifunctional protein glmU releasing pyrophosphate and a Uridine diphosphate-N-acetylglucosamine.This compound can either be isomerized into a UDP-N-acetyl-D-mannosamine or undergo a reaction with phosphoenolpyruvic acid through UDP-N-acetylglucosamine 1-carboxyvinyltransferase releasing a phosphate and a UDP-N-Acetyl-alpha-D-glucosamine-enolpyruvate.
UDP-N-acetyl-D-mannosamine undergoes a NAD dependent dehydrogenation through a UDP-N-acetyl-D-mannosamine dehydrogenase, releasing NADH, a hydrogen ion and a UDP-N-Acetyl-alpha-D-mannosaminuronate, This compound is then used in the production of enterobacterial common antigens.
UDP-N-Acetyl-alpha-D-glucosamine-enolpyruvate is reduced through a NADPH dependent UDP-N-acetylenolpyruvoylglucosamine reductase, releasing a NADP and a UDP-N-acetyl-alpha-D-muramate. The latter is also involved in the D-glutamine and D-glutamate metabolism.
|
Metabolite
Metabolic
|
|
|
SMP0343737 |
Cardiolipin Biosynthesis CL(20:4(5Z,8Z,11Z,14Z)/22:6(4Z,7Z,10Z,13Z,16Z,19Z)/16:1(9Z)/16:1(9Z))Mus musculus
Cardiolipin (CL) is an important component of the inner mitochondrial membrane where it constitutes about 20% of the total lipid composition. It is essential for the optimal function of numerous enzymes that are involved in mitochondrial energy metabolism (Wikipedia). Cardiolipin biosynthesis occurs mainly in the mitochondria, but there also exists an alternative synthesis route for CDP-diacylglycerol that takes place in the endoplasmic reticulum. This second route may supplement this pathway. All membrane-localized enzymes are coloured dark green in the image. First, dihydroxyacetone phosphate (or glycerone phosphate) from glycolysis is used by the cytosolic enzyme glycerol-3-phosphate dehydrogenase [NAD(+)] to synthesize sn-glycerol 3-phosphate. Second, the mitochondrial outer membrane enzyme glycerol-3-phosphate acyltransferase esterifies an acyl-group to the sn-1 position of sn-glycerol 3-phosphate to form 1-acyl-sn-glycerol 3-phosphate (lysophosphatidic acid or LPA). Third, the enzyme 1-acyl-sn-glycerol-3-phosphate acyltransferase converts LPA into phosphatidic acid (PA or 1,2-diacyl-sn-glycerol 3-phosphate) by esterifying an acyl-group to the sn-2 position of the glycerol backbone. PA is then transferred to the inner mitochondrial membrane to continue cardiolipin synthesis. Fourth, magnesium-dependent phosphatidate cytidylyltransferase catalyzes the conversion of PA into CDP-diacylglycerol. Fifth, CDP-diacylglycerol--glycerol-3-phosphate 3-phosphatidyltransferase synthesizes phosphatidylglycerophosphate (PGP). Sixth, phosphatidylglycerophosphatase and protein-tyrosine phosphatase dephosphorylates PGP to form phosphatidylglycerol (PG). Last, cardiolipin synthase catalyzes the synthesis of cardiolipin by transferring a phosphatidyl group from a second CDP-diacylglycerol to PG. It requires a divalent metal cation cofactor.
|
Metabolite
Metabolic
|
|
|
SMP0449051![]() |
Propanoate MetabolismRaoultella planticola
Starting from L-threonine, this compound is deaminated through a threonine deaminase resulting in a hydrogen ion, a water molecule and a (2z)-2-aminobut-2-enoate. The latter compound then isomerizes to a 2-iminobutanoate, This compound then reacts spontaneously with hydrogen ion and a water molecule resulting in a ammonium and a 2-Ketobutyric acid. The latter compound interacts with CoA through a pyruvate formate-lyase / 2-ketobutyrate formate-lyase resulting in a formic acid and a propionyl-CoA.
Propionyl-CoA can then be processed either into a 2-methylcitric acid or into a propanoyl phosphate. Propionyl-CoA interacts with oxalacetic acid and a water molecule through a 2-methylcitrate synthase resulting in a hydrogen ion, a CoA and a 2-Methylcitric acid.The latter compound is dehydrated through a 2-methylcitrate dehydratase resulting in a water molecule and cis-2-methylaconitate. The latter compound is then dehydrated by a bifunctional aconitate hydratase 2 and 2-methylisocitrate dehydratase resulting in a water molecule and methylisocitric acid. The latter compound is then processed by 2-methylisocitrate lyase resulting in a release of succinic acid and pyruvic acid. Succinic acid can then interact with a propionyl-CoA through a propionyl-CoA:succinate CoA transferase resulting in a propionic acid and a succinyl CoA. Succinyl-CoA is then isomerized through a methylmalonyl-CoA mutase resulting in a methylmalonyl-CoA. This compound is then decarboxylated through a methylmalonyl-CoA decarboxylase resulting in a release of Carbon dioxide and Propionyl-CoA. Propionyl-CoA interacts with a phosphate through a phosphate acetyltransferase / phosphate propionyltransferase resulting in a CoA and a propanoyl phosphate. Propionyl-CoA can react with a phosphate through a phosphate acetyltransferase / phosphate propionyltransferase resulting in a CoA and a propanoyl phosphate. The latter compound is then dephosphorylated through a ADP driven acetate kinase/propionate kinase protein complex resulting in an ATP and Propionic acid. Propionic acid can be processed by a reaction with CoA through a ATP-driven propionyl-CoA synthetase resulting in a pyrophosphate, an AMP and a propionyl-CoA.
|
Metabolite
Metabolic
|
|
|
SMP0449042![]() |
Galactose Degradation/Leloir PathwayEscherichia coli IAI39
The degradation of galactose, also known as Leloir pathway, requires 3 main enzymes once Beta-D-galactose has been converted to galactose through an Aldose-1-epimerase. These are: galactokinase , galactose-1-phosphate uridylyltransferase and UDP-glucose 4-epimerase. Beta-D-galactose can be uptaken from the environment through a galactose proton symporter. It can also be produced by lactose degradation involving a lactose permease to uptake lactose from the environment and a beta-galactosidase to turn lactose into Beta-D-galactose.
Galactose is degraded through the following process:
Beta-D-galactose is introduced into the cytoplasm through a galactose proton symporter, or it can be synthesized from an alpha lactose that is introduced into the cytoplasm through a lactose permease. Alpha lactose interacts with water through a beta-galactosidase resulting in a beta-D-glucose and beta-D-galactose. Beta-D-galactose is isomerized into D-galactose. D-Galactose undergoes phosphorylation through a galactokinase, hence producing galactose 1 phosphate. On the other side of the pathway, a gluose-1-phosphate (product of the interaction of alpha-D-glucose 6-phosphate with a phosphoglucomutase resulting in a alpha-D-glucose-1-phosphate, an isomer of Glucose 1-phosphate, or an isomer of Beta-D-glucose 1-phosphate) interacts with UTP and a hydrogen ion in order to produce a uridine diphosphate glucose. This is followed by the interaction of galactose-1-phosphate with an established amount of uridine diphosphate glucose through a galactose-1-phosphate uridylyltransferase, which in turn output a glucose-1-phosphate and a uridine diphosphate galactose. The glucose -1-phosphate is transformed into a uridine diphosphate glucose through UTP--glucose-1-phosphate uridylyltransferase. The product, uridine diphosphate glucose, can undergo a reversible reaction in which it can be turned into uridine diphosphategalactose through an UDP-glucose 4-epimerase, and so the cycle can keep going as long as more lactose or galactose is imported into the cell.
|
Metabolite
Metabolic
|
|
|
SMP0343732 |
Cardiolipin Biosynthesis CL(20:4(5Z,8Z,11Z,14Z)/22:6(4Z,7Z,10Z,13Z,16Z,19Z)/16:0/22:5(4Z,7Z,10Z,13Z,16Z))Mus musculus
Cardiolipin (CL) is an important component of the inner mitochondrial membrane where it constitutes about 20% of the total lipid composition. It is essential for the optimal function of numerous enzymes that are involved in mitochondrial energy metabolism (Wikipedia). Cardiolipin biosynthesis occurs mainly in the mitochondria, but there also exists an alternative synthesis route for CDP-diacylglycerol that takes place in the endoplasmic reticulum. This second route may supplement this pathway. All membrane-localized enzymes are coloured dark green in the image. First, dihydroxyacetone phosphate (or glycerone phosphate) from glycolysis is used by the cytosolic enzyme glycerol-3-phosphate dehydrogenase [NAD(+)] to synthesize sn-glycerol 3-phosphate. Second, the mitochondrial outer membrane enzyme glycerol-3-phosphate acyltransferase esterifies an acyl-group to the sn-1 position of sn-glycerol 3-phosphate to form 1-acyl-sn-glycerol 3-phosphate (lysophosphatidic acid or LPA). Third, the enzyme 1-acyl-sn-glycerol-3-phosphate acyltransferase converts LPA into phosphatidic acid (PA or 1,2-diacyl-sn-glycerol 3-phosphate) by esterifying an acyl-group to the sn-2 position of the glycerol backbone. PA is then transferred to the inner mitochondrial membrane to continue cardiolipin synthesis. Fourth, magnesium-dependent phosphatidate cytidylyltransferase catalyzes the conversion of PA into CDP-diacylglycerol. Fifth, CDP-diacylglycerol--glycerol-3-phosphate 3-phosphatidyltransferase synthesizes phosphatidylglycerophosphate (PGP). Sixth, phosphatidylglycerophosphatase and protein-tyrosine phosphatase dephosphorylates PGP to form phosphatidylglycerol (PG). Last, cardiolipin synthase catalyzes the synthesis of cardiolipin by transferring a phosphatidyl group from a second CDP-diacylglycerol to PG. It requires a divalent metal cation cofactor.
|
Metabolite
Metabolic
|
|
Showing 371721 -
371730 of 372053 pathways