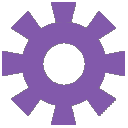
Browsing Pathways
Showing 372421 -
372430 of 605359 pathways
PathBank ID | Pathway Name and Description | Pathway Class | Chemical Compounds | Proteins |
---|---|---|---|---|
SMP0350490 |
Cardiolipin Biosynthesis CL(i-12:0/a-13:0/16:0/i-16:0)Homo sapiens
Cardiolipin (CL) is an important component of the inner mitochondrial membrane where it constitutes about 20% of the total lipid composition. It is essential for the optimal function of numerous enzymes that are involved in mitochondrial energy metabolism (Wikipedia). Cardiolipin biosynthesis occurs mainly in the mitochondria, but there also exists an alternative synthesis route for CDP-diacylglycerol that takes place in the endoplasmic reticulum. This second route may supplement this pathway. All membrane-localized enzymes are coloured dark green in the image. First, dihydroxyacetone phosphate (or glycerone phosphate) from glycolysis is used by the cytosolic enzyme glycerol-3-phosphate dehydrogenase [NAD(+)] to synthesize sn-glycerol 3-phosphate. Second, the mitochondrial outer membrane enzyme glycerol-3-phosphate acyltransferase esterifies an acyl-group to the sn-1 position of sn-glycerol 3-phosphate to form 1-acyl-sn-glycerol 3-phosphate (lysophosphatidic acid or LPA). Third, the enzyme 1-acyl-sn-glycerol-3-phosphate acyltransferase converts LPA into phosphatidic acid (PA or 1,2-diacyl-sn-glycerol 3-phosphate) by esterifying an acyl-group to the sn-2 position of the glycerol backbone. PA is then transferred to the inner mitochondrial membrane to continue cardiolipin synthesis. Fourth, magnesium-dependent phosphatidate cytidylyltransferase catalyzes the conversion of PA into CDP-diacylglycerol. Fifth, CDP-diacylglycerol--glycerol-3-phosphate 3-phosphatidyltransferase synthesizes phosphatidylglycerophosphate (PGP). Sixth, phosphatidylglycerophosphatase and protein-tyrosine phosphatase dephosphorylates PGP to form phosphatidylglycerol (PG). Last, cardiolipin synthase catalyzes the synthesis of cardiolipin by transferring a phosphatidyl group from a second CDP-diacylglycerol to PG. It requires a divalent metal cation cofactor.
|
Metabolite
Metabolic
|
|
|
SMP0350478 |
Cardiolipin Biosynthesis CL(i-12:0/a-13:0/16:0/19:0)Homo sapiens
Cardiolipin (CL) is an important component of the inner mitochondrial membrane where it constitutes about 20% of the total lipid composition. It is essential for the optimal function of numerous enzymes that are involved in mitochondrial energy metabolism (Wikipedia). Cardiolipin biosynthesis occurs mainly in the mitochondria, but there also exists an alternative synthesis route for CDP-diacylglycerol that takes place in the endoplasmic reticulum. This second route may supplement this pathway. All membrane-localized enzymes are coloured dark green in the image. First, dihydroxyacetone phosphate (or glycerone phosphate) from glycolysis is used by the cytosolic enzyme glycerol-3-phosphate dehydrogenase [NAD(+)] to synthesize sn-glycerol 3-phosphate. Second, the mitochondrial outer membrane enzyme glycerol-3-phosphate acyltransferase esterifies an acyl-group to the sn-1 position of sn-glycerol 3-phosphate to form 1-acyl-sn-glycerol 3-phosphate (lysophosphatidic acid or LPA). Third, the enzyme 1-acyl-sn-glycerol-3-phosphate acyltransferase converts LPA into phosphatidic acid (PA or 1,2-diacyl-sn-glycerol 3-phosphate) by esterifying an acyl-group to the sn-2 position of the glycerol backbone. PA is then transferred to the inner mitochondrial membrane to continue cardiolipin synthesis. Fourth, magnesium-dependent phosphatidate cytidylyltransferase catalyzes the conversion of PA into CDP-diacylglycerol. Fifth, CDP-diacylglycerol--glycerol-3-phosphate 3-phosphatidyltransferase synthesizes phosphatidylglycerophosphate (PGP). Sixth, phosphatidylglycerophosphatase and protein-tyrosine phosphatase dephosphorylates PGP to form phosphatidylglycerol (PG). Last, cardiolipin synthase catalyzes the synthesis of cardiolipin by transferring a phosphatidyl group from a second CDP-diacylglycerol to PG. It requires a divalent metal cation cofactor.
|
Metabolite
Metabolic
|
|
|
SMP0350485 |
Cardiolipin Biosynthesis CL(i-12:0/a-13:0/16:0/25:0)Homo sapiens
Cardiolipin (CL) is an important component of the inner mitochondrial membrane where it constitutes about 20% of the total lipid composition. It is essential for the optimal function of numerous enzymes that are involved in mitochondrial energy metabolism (Wikipedia). Cardiolipin biosynthesis occurs mainly in the mitochondria, but there also exists an alternative synthesis route for CDP-diacylglycerol that takes place in the endoplasmic reticulum. This second route may supplement this pathway. All membrane-localized enzymes are coloured dark green in the image. First, dihydroxyacetone phosphate (or glycerone phosphate) from glycolysis is used by the cytosolic enzyme glycerol-3-phosphate dehydrogenase [NAD(+)] to synthesize sn-glycerol 3-phosphate. Second, the mitochondrial outer membrane enzyme glycerol-3-phosphate acyltransferase esterifies an acyl-group to the sn-1 position of sn-glycerol 3-phosphate to form 1-acyl-sn-glycerol 3-phosphate (lysophosphatidic acid or LPA). Third, the enzyme 1-acyl-sn-glycerol-3-phosphate acyltransferase converts LPA into phosphatidic acid (PA or 1,2-diacyl-sn-glycerol 3-phosphate) by esterifying an acyl-group to the sn-2 position of the glycerol backbone. PA is then transferred to the inner mitochondrial membrane to continue cardiolipin synthesis. Fourth, magnesium-dependent phosphatidate cytidylyltransferase catalyzes the conversion of PA into CDP-diacylglycerol. Fifth, CDP-diacylglycerol--glycerol-3-phosphate 3-phosphatidyltransferase synthesizes phosphatidylglycerophosphate (PGP). Sixth, phosphatidylglycerophosphatase and protein-tyrosine phosphatase dephosphorylates PGP to form phosphatidylglycerol (PG). Last, cardiolipin synthase catalyzes the synthesis of cardiolipin by transferring a phosphatidyl group from a second CDP-diacylglycerol to PG. It requires a divalent metal cation cofactor.
|
Metabolite
Metabolic
|
|
|
SMP0453393![]() |
GTP Degradation and Molybdenum Cofactor BiosynthesisEscherichia coli O26:H11 str. 11368
GTP, produced in the nucleotide de novo biosyntheis pathway, interacts with a water molecule through a GTP cyclohydrolase resulting in a formate, hydrogen ion and a 7,8-dihydroneopterin 3'-triphosphate. The latter compound interacts with a water molecule through a dihydroneopterin triphosphate pyrophosphohydrolase resulting in the release of a pyrophosphate, a hydrogen ion and a 7,8-dihydroneopterin 3'-phosphate. The latter compound interacts with water spontaneously resulting in the release of a phosphate and a 7,8 dihydroneopterin. The latter compound interacts with a dihydroneopterin aldolase resulting in the release of a glycolaldehyde and a 6-hydroxymethyl-7,8-dihydropterin. This compound then is then diphosphorylated by reacting with a ATP driven 6-hydroxymethyl-7,8-dihydropterin pyrophosphokinase resulting in the release of a hydrogen ion, an AMP and 6-hydroxymethyl-7,8-dihydropterin diphosphate.
GTP interacts with a cyclic pyranopterin monophosphate synthase resulting in the release of a diphosphate and a cyclic pyranopterin phosphate. The latter compound interacts with a thiocarboxylated small subunit of molybdopterin synthase (a protein) and a water molecule through a molybdopterin synthase resulting in the release of 4 hydrogen ions, 2 small subunits of molybdopterin synthase and a molybdopterin. The molybdopterin interacts with an ATP and a hydrogen ion through a molybdopterin adenylyltransferase resulting in the release of a diphosphate and a molybdopterin adenine dinucleotide. The latter compound is then metabolized by a hydrogen ion and a molybdate through a molybdopterin molybdenumtransferase resulting in the release of an AMP, a water molecule and a molybdopterin cofactor.
The molybdopterin cofactor can procede to the guanylyl molybdenum cofactor biosynthesis pathway or it can be metabolized into a cytidylyl molybdenum cofactor by interacting with a CTP and a hydrogen ion through a molybdenym cofactor cytidylyltransferase resulting in the release of a pyrophosphate and a cytidyllyl molybdenum cofactor
|
Metabolite
Metabolic
|
|
|
SMP0453388![]() |
GTP Degradation and Molybdenum Cofactor BiosynthesisEscherichia coli O103:H2 str. 12009
GTP, produced in the nucleotide de novo biosyntheis pathway, interacts with a water molecule through a GTP cyclohydrolase resulting in a formate, hydrogen ion and a 7,8-dihydroneopterin 3'-triphosphate. The latter compound interacts with a water molecule through a dihydroneopterin triphosphate pyrophosphohydrolase resulting in the release of a pyrophosphate, a hydrogen ion and a 7,8-dihydroneopterin 3'-phosphate. The latter compound interacts with water spontaneously resulting in the release of a phosphate and a 7,8 dihydroneopterin. The latter compound interacts with a dihydroneopterin aldolase resulting in the release of a glycolaldehyde and a 6-hydroxymethyl-7,8-dihydropterin. This compound then is then diphosphorylated by reacting with a ATP driven 6-hydroxymethyl-7,8-dihydropterin pyrophosphokinase resulting in the release of a hydrogen ion, an AMP and 6-hydroxymethyl-7,8-dihydropterin diphosphate.
GTP interacts with a cyclic pyranopterin monophosphate synthase resulting in the release of a diphosphate and a cyclic pyranopterin phosphate. The latter compound interacts with a thiocarboxylated small subunit of molybdopterin synthase (a protein) and a water molecule through a molybdopterin synthase resulting in the release of 4 hydrogen ions, 2 small subunits of molybdopterin synthase and a molybdopterin. The molybdopterin interacts with an ATP and a hydrogen ion through a molybdopterin adenylyltransferase resulting in the release of a diphosphate and a molybdopterin adenine dinucleotide. The latter compound is then metabolized by a hydrogen ion and a molybdate through a molybdopterin molybdenumtransferase resulting in the release of an AMP, a water molecule and a molybdopterin cofactor.
The molybdopterin cofactor can procede to the guanylyl molybdenum cofactor biosynthesis pathway or it can be metabolized into a cytidylyl molybdenum cofactor by interacting with a CTP and a hydrogen ion through a molybdenym cofactor cytidylyltransferase resulting in the release of a pyrophosphate and a cytidyllyl molybdenum cofactor
|
Metabolite
Metabolic
|
|
|
SMP0350593 |
Cardiolipin Biosynthesis CL(i-12:0/a-13:0/21:0/25:0)Homo sapiens
Cardiolipin (CL) is an important component of the inner mitochondrial membrane where it constitutes about 20% of the total lipid composition. It is essential for the optimal function of numerous enzymes that are involved in mitochondrial energy metabolism (Wikipedia). Cardiolipin biosynthesis occurs mainly in the mitochondria, but there also exists an alternative synthesis route for CDP-diacylglycerol that takes place in the endoplasmic reticulum. This second route may supplement this pathway. All membrane-localized enzymes are coloured dark green in the image. First, dihydroxyacetone phosphate (or glycerone phosphate) from glycolysis is used by the cytosolic enzyme glycerol-3-phosphate dehydrogenase [NAD(+)] to synthesize sn-glycerol 3-phosphate. Second, the mitochondrial outer membrane enzyme glycerol-3-phosphate acyltransferase esterifies an acyl-group to the sn-1 position of sn-glycerol 3-phosphate to form 1-acyl-sn-glycerol 3-phosphate (lysophosphatidic acid or LPA). Third, the enzyme 1-acyl-sn-glycerol-3-phosphate acyltransferase converts LPA into phosphatidic acid (PA or 1,2-diacyl-sn-glycerol 3-phosphate) by esterifying an acyl-group to the sn-2 position of the glycerol backbone. PA is then transferred to the inner mitochondrial membrane to continue cardiolipin synthesis. Fourth, magnesium-dependent phosphatidate cytidylyltransferase catalyzes the conversion of PA into CDP-diacylglycerol. Fifth, CDP-diacylglycerol--glycerol-3-phosphate 3-phosphatidyltransferase synthesizes phosphatidylglycerophosphate (PGP). Sixth, phosphatidylglycerophosphatase and protein-tyrosine phosphatase dephosphorylates PGP to form phosphatidylglycerol (PG). Last, cardiolipin synthase catalyzes the synthesis of cardiolipin by transferring a phosphatidyl group from a second CDP-diacylglycerol to PG. It requires a divalent metal cation cofactor.
|
Metabolite
Metabolic
|
|
|
SMP0453571![]() |
Galactose Degradation/Leloir PathwayPaenibacillus lactis 154
The degradation of galactose, also known as Leloir pathway, requires 3 main enzymes once Beta-D-galactose has been converted to galactose through an Aldose-1-epimerase. These are: galactokinase , galactose-1-phosphate uridylyltransferase and UDP-glucose 4-epimerase. Beta-D-galactose can be uptaken from the environment through a galactose proton symporter. It can also be produced by lactose degradation involving a lactose permease to uptake lactose from the environment and a beta-galactosidase to turn lactose into Beta-D-galactose.
Galactose is degraded through the following process:
Beta-D-galactose is introduced into the cytoplasm through a galactose proton symporter, or it can be synthesized from an alpha lactose that is introduced into the cytoplasm through a lactose permease. Alpha lactose interacts with water through a beta-galactosidase resulting in a beta-D-glucose and beta-D-galactose. Beta-D-galactose is isomerized into D-galactose. D-Galactose undergoes phosphorylation through a galactokinase, hence producing galactose 1 phosphate. On the other side of the pathway, a gluose-1-phosphate (product of the interaction of alpha-D-glucose 6-phosphate with a phosphoglucomutase resulting in a alpha-D-glucose-1-phosphate, an isomer of Glucose 1-phosphate, or an isomer of Beta-D-glucose 1-phosphate) interacts with UTP and a hydrogen ion in order to produce a uridine diphosphate glucose. This is followed by the interaction of galactose-1-phosphate with an established amount of uridine diphosphate glucose through a galactose-1-phosphate uridylyltransferase, which in turn output a glucose-1-phosphate and a uridine diphosphate galactose. The glucose -1-phosphate is transformed into a uridine diphosphate glucose through UTP--glucose-1-phosphate uridylyltransferase. The product, uridine diphosphate glucose, can undergo a reversible reaction in which it can be turned into uridine diphosphategalactose through an UDP-glucose 4-epimerase, and so the cycle can keep going as long as more lactose or galactose is imported into the cell.
|
Metabolite
Metabolic
|
|
|
SMP0453554![]() |
Adenosylcobalamin Salvage from CobinamideKlebsiella pneumoniae subsp. pneumoniae MGH 78578
Cobinamide is incorporated from the extracellular space through a transport system into the cytosol. Once inside the cytosol, cobinamide interacts with ATP through a cobinamide adenosyl transferase resulting in the release of a triphosphate and an adenosylcobinamide. The latter compound is then phosphorylated through an ATP-dependent cobinamide kinase resulting in the release of ADP, a hydrogen ion and adenosyl-cobinamide phosphate. This last compound then interacts with GTP and a hydrogen ion through a cobinamide-P guanylyltransferase resulting in the release of a pyrophosphate and an adenosylcobinamide-GDP.
A dimethylbenzimidazole interacts with a nicotinate D-ribonucleotide through a nicotinate-nucleotide dimethylbenzumidazole phosphoribosyltransferase resulting in the release of a nicotinate, a hydrogen ion and an alpha-ribazole 5' phosphate.
The adenosylcobinamide-GDP and the alpha-ribazole 5' phosphate interact together through a cobalamin 5' phosphate synthase resulting in the release of a hydrogen ion, a GMP and Adenosylcobalamin 5'-phosphate. The latter compound then interacts with a water molecule through an adenosylcbalamin 5' phosphate phosphatase resulting in the release of a phosphate and a coenzyme B12.
Likewise a cobalamin molecule can interact with ATP through a cobalamin adenosyltransferase resulting in the release of a triphosphate and a coenzyme B12
|
Metabolite
Metabolic
|
|
|
SMP0453559![]() |
Adenosylcobalamin Salvage from CobinamideLaribacter hongkongensis HLHK9
Cobinamide is incorporated from the extracellular space through a transport system into the cytosol. Once inside the cytosol, cobinamide interacts with ATP through a cobinamide adenosyl transferase resulting in the release of a triphosphate and an adenosylcobinamide. The latter compound is then phosphorylated through an ATP-dependent cobinamide kinase resulting in the release of ADP, a hydrogen ion and adenosyl-cobinamide phosphate. This last compound then interacts with GTP and a hydrogen ion through a cobinamide-P guanylyltransferase resulting in the release of a pyrophosphate and an adenosylcobinamide-GDP.
A dimethylbenzimidazole interacts with a nicotinate D-ribonucleotide through a nicotinate-nucleotide dimethylbenzumidazole phosphoribosyltransferase resulting in the release of a nicotinate, a hydrogen ion and an alpha-ribazole 5' phosphate.
The adenosylcobinamide-GDP and the alpha-ribazole 5' phosphate interact together through a cobalamin 5' phosphate synthase resulting in the release of a hydrogen ion, a GMP and Adenosylcobalamin 5'-phosphate. The latter compound then interacts with a water molecule through an adenosylcbalamin 5' phosphate phosphatase resulting in the release of a phosphate and a coenzyme B12.
Likewise a cobalamin molecule can interact with ATP through a cobalamin adenosyltransferase resulting in the release of a triphosphate and a coenzyme B12
|
Metabolite
Metabolic
|
|
|
SMP0453560![]() |
Folate BiosynthesisPyramidobacter piscolens W5455
The biosynthesis of folic acid begins as a product of purine nucleotides de novo biosynthesis pathway. Purine nucleotides are involved in a reaction with water through a GTP cyclohydrolase 1 protein complex, resulting in a hydrogen ion, formic acid and 7,8-dihydroneopterin 3-triphosphate. The latter compound is dephosphorylated through a dihydroneopterin triphosphate pyrophosphohydrolase resulting in the release of a pyrophosphate, hydrogen ion and 7,8-dihydroneopterin 3-phosphate. The latter product reacts with water spontaneously resulting in the release of a phosphate and a 7,8 -dihydroneopterin. 7,8 -dihydroneopterin reacts with a dihydroneopterin aldolase, releasing a glycoaldehyde and 6-hydroxymethyl-7,9-dihydropterin. Continuing, 6-hydroxymethyl-7,9-dihydropterin is phosphorylated with a ATP-driven 6-hydroxymethyl-7,8-dihydropterin pyrophosphokinase resulting in a (2-amino-4-hydroxy-7,8-dihydropteridin-6-yl)methyl diphosphate.
Chorismate is metabolized by reacting with L-glutamine through a 4-amino-4-deoxychorismate synthase resulting in L-glutamic acid and 4-amino-4-deoxychorismate. The latter product is then catalyzed via an aminodeoxychorismate lyase resulting in pyruvic acid, hydrogen ion and p-aminobenzoic acid.
(2-amino-4-hydroxy-7,8-dihydropteridin-6-yl)methyl diphosphate and p-aminobenzoic acid react with the help of a dihydropteroate synthase resulting in pyrophosphate and 7,8-dihydropteroic acid. This compound then reacts with L-glutamic acid through an ATP driven bifunctional folylpolyglutamate synthease / dihydrofolate synthease resulting in a 7,8-dihydrofolate monoglutamate. 7,8-dihydrofolate monoglutamate is then reduced via a NADPH mediated dihydrofolate reductase resulting in a tetrahydrofate which will continue and become a metabolite of the folate pathway
|
Metabolite
Metabolic
|
|
Showing 372421 -
372430 of 372738 pathways