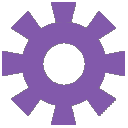
Browsing Pathways
Showing 372441 -
372450 of 605359 pathways
PathBank ID | Pathway Name and Description | Pathway Class | Chemical Compounds | Proteins |
---|---|---|---|---|
SMP0452039![]() |
Glutathione MetabolismCampylobacter jejuni subsp. jejuni ICDCCJ07004
The biosynthesis of glutathione starts with the introduction of L-glutamic acid through either a glutamate:sodium symporter, glutamate / aspartate : H+ symporter GltP or a
glutamate / aspartate ABC transporter. Once in the cytoplasm, L-glutamice acid reacts with L-cysteine through an ATP glutamate-cysteine ligase resulting in gamma-glutamylcysteine. This compound reacts which Glycine through an ATP driven glutathione synthetase thus catabolizing Glutathione.
This compound is metabolized through a spontaneous reaction with an oxidized glutaredoxin resulting in a reduced glutaredoxin and an oxidized glutathione. This compound is reduced by a NADPH glutathione reductase resulting in a glutathione.
Glutathione can then be degraded into various different glutathione containing compounds by reacting with a napthalene or Bromobenzene-2,3-oxide through a glutathione S-transferase.
|
Metabolite
Metabolic
|
|
|
SMP0347370 |
Cardiolipin Biosynthesis CL(8:0/a-13:0/14:0/i-22:0)Homo sapiens
Cardiolipin (CL) is an important component of the inner mitochondrial membrane where it constitutes about 20% of the total lipid composition. It is essential for the optimal function of numerous enzymes that are involved in mitochondrial energy metabolism (Wikipedia). Cardiolipin biosynthesis occurs mainly in the mitochondria, but there also exists an alternative synthesis route for CDP-diacylglycerol that takes place in the endoplasmic reticulum. This second route may supplement this pathway. All membrane-localized enzymes are coloured dark green in the image. First, dihydroxyacetone phosphate (or glycerone phosphate) from glycolysis is used by the cytosolic enzyme glycerol-3-phosphate dehydrogenase [NAD(+)] to synthesize sn-glycerol 3-phosphate. Second, the mitochondrial outer membrane enzyme glycerol-3-phosphate acyltransferase esterifies an acyl-group to the sn-1 position of sn-glycerol 3-phosphate to form 1-acyl-sn-glycerol 3-phosphate (lysophosphatidic acid or LPA). Third, the enzyme 1-acyl-sn-glycerol-3-phosphate acyltransferase converts LPA into phosphatidic acid (PA or 1,2-diacyl-sn-glycerol 3-phosphate) by esterifying an acyl-group to the sn-2 position of the glycerol backbone. PA is then transferred to the inner mitochondrial membrane to continue cardiolipin synthesis. Fourth, magnesium-dependent phosphatidate cytidylyltransferase catalyzes the conversion of PA into CDP-diacylglycerol. Fifth, CDP-diacylglycerol--glycerol-3-phosphate 3-phosphatidyltransferase synthesizes phosphatidylglycerophosphate (PGP). Sixth, phosphatidylglycerophosphatase and protein-tyrosine phosphatase dephosphorylates PGP to form phosphatidylglycerol (PG). Last, cardiolipin synthase catalyzes the synthesis of cardiolipin by transferring a phosphatidyl group from a second CDP-diacylglycerol to PG. It requires a divalent metal cation cofactor.
|
Metabolite
Metabolic
|
|
|
SMP0347412 |
Cardiolipin Biosynthesis CL(8:0/a-13:0/15:0/17:0)Homo sapiens
Cardiolipin (CL) is an important component of the inner mitochondrial membrane where it constitutes about 20% of the total lipid composition. It is essential for the optimal function of numerous enzymes that are involved in mitochondrial energy metabolism (Wikipedia). Cardiolipin biosynthesis occurs mainly in the mitochondria, but there also exists an alternative synthesis route for CDP-diacylglycerol that takes place in the endoplasmic reticulum. This second route may supplement this pathway. All membrane-localized enzymes are coloured dark green in the image. First, dihydroxyacetone phosphate (or glycerone phosphate) from glycolysis is used by the cytosolic enzyme glycerol-3-phosphate dehydrogenase [NAD(+)] to synthesize sn-glycerol 3-phosphate. Second, the mitochondrial outer membrane enzyme glycerol-3-phosphate acyltransferase esterifies an acyl-group to the sn-1 position of sn-glycerol 3-phosphate to form 1-acyl-sn-glycerol 3-phosphate (lysophosphatidic acid or LPA). Third, the enzyme 1-acyl-sn-glycerol-3-phosphate acyltransferase converts LPA into phosphatidic acid (PA or 1,2-diacyl-sn-glycerol 3-phosphate) by esterifying an acyl-group to the sn-2 position of the glycerol backbone. PA is then transferred to the inner mitochondrial membrane to continue cardiolipin synthesis. Fourth, magnesium-dependent phosphatidate cytidylyltransferase catalyzes the conversion of PA into CDP-diacylglycerol. Fifth, CDP-diacylglycerol--glycerol-3-phosphate 3-phosphatidyltransferase synthesizes phosphatidylglycerophosphate (PGP). Sixth, phosphatidylglycerophosphatase and protein-tyrosine phosphatase dephosphorylates PGP to form phosphatidylglycerol (PG). Last, cardiolipin synthase catalyzes the synthesis of cardiolipin by transferring a phosphatidyl group from a second CDP-diacylglycerol to PG. It requires a divalent metal cation cofactor.
|
Metabolite
Metabolic
|
|
|
SMP0452045![]() |
Galactose Degradation/Leloir PathwayCampylobacter jejuni subsp. jejuni ICDCCJ07004
The degradation of galactose, also known as Leloir pathway, requires 3 main enzymes once Beta-D-galactose has been converted to galactose through an Aldose-1-epimerase. These are: galactokinase , galactose-1-phosphate uridylyltransferase and UDP-glucose 4-epimerase. Beta-D-galactose can be uptaken from the environment through a galactose proton symporter. It can also be produced by lactose degradation involving a lactose permease to uptake lactose from the environment and a beta-galactosidase to turn lactose into Beta-D-galactose.
Galactose is degraded through the following process:
Beta-D-galactose is introduced into the cytoplasm through a galactose proton symporter, or it can be synthesized from an alpha lactose that is introduced into the cytoplasm through a lactose permease. Alpha lactose interacts with water through a beta-galactosidase resulting in a beta-D-glucose and beta-D-galactose. Beta-D-galactose is isomerized into D-galactose. D-Galactose undergoes phosphorylation through a galactokinase, hence producing galactose 1 phosphate. On the other side of the pathway, a gluose-1-phosphate (product of the interaction of alpha-D-glucose 6-phosphate with a phosphoglucomutase resulting in a alpha-D-glucose-1-phosphate, an isomer of Glucose 1-phosphate, or an isomer of Beta-D-glucose 1-phosphate) interacts with UTP and a hydrogen ion in order to produce a uridine diphosphate glucose. This is followed by the interaction of galactose-1-phosphate with an established amount of uridine diphosphate glucose through a galactose-1-phosphate uridylyltransferase, which in turn output a glucose-1-phosphate and a uridine diphosphate galactose. The glucose -1-phosphate is transformed into a uridine diphosphate glucose through UTP--glucose-1-phosphate uridylyltransferase. The product, uridine diphosphate glucose, can undergo a reversible reaction in which it can be turned into uridine diphosphategalactose through an UDP-glucose 4-epimerase, and so the cycle can keep going as long as more lactose or galactose is imported into the cell.
|
Metabolite
Metabolic
|
|
|
SMP0452034![]() |
Phospholipid BiosynthesisBacteroides fragilis NCTC 9343
Phospholipids are membrane components in E. coli. The major phospholipids of E. coli are phosphatidylethanolamine, phosphatidylglycerol, and cardiolipin. All phospholipids contain sn-glycerol-3-phosphate esterified with fatty acids at the sn-1 and sn-2 positions. The reaction starts from a glycerone phosphate (dihydroxyacetone phosphate) produced in glycolysis. The glycerone phosphate is transformed into an sn-glycerol 3-phosphate (glycerol 3 phosphate) by NADPH-driven glycerol-3-phosphate dehydrogenase. sn-Glycerol 3-phosphate is transformed to a 1-acyl-sn-glycerol 3-phosphate (lysophosphatidic acid). This can be achieved by an sn-glycerol-3-phosphate acyltransferase that interacts either with a long-chain acyl-CoA or with an acyl-[acp]. The 1-acyl-sn-glycerol 3-phosphate is transformed into a 1,2-diacyl-sn-glycerol 3-phosphate (phosphatidic acid) through a 1-acylglycerol-3-phosphate O-acyltransferase. This compound is then converted into a CPD-diacylglycerol through a CTP phosphatidate cytididyltransferase. CPD-diacylglycerol can be transformed either into an L-1-phosphatidylserine or an L-1-phosphatidylglycerol-phosphate through a phosphatidylserine synthase or a phosphatidylglycerophosphate synthase, respectively. The L-1-phosphatidylserine transforms into L-1-phosphatidylethanolamine through a phosphatidylserine decarboxylase. On the other hand, L-1-phosphatidylglycerol-phosphate gets transformed into an L-1-phosphatidyl-glycerol through a phosphatidylglycerophosphatase. These 2 products combine to produce a cardiolipin and an ethanolamine. The L-1 phosphatidyl-glycerol can also interact with cardiolipin synthase resulting in a glycerol and a cardiolipin.
|
Metabolite
Metabolic
|
||
SMP0452050![]() |
Purine DegradationBacteroides fragilis NCTC 9343
Pseudouridine is phosphorylated by interacting with atp and a psuK resulting in the release of an ADP, a hydrogen ion and a pseudouridine 5'-phosphate. The latter compound then reacts with water through a pseudouridine 5'-phosphate glycosidase resulting in the release of a uracil and D-ribofuranose 5-phosphate
|
Metabolite
Metabolic
|
||
SMP0452196![]() |
Secondary Metabolites: Ubiquinol BiosynthesisEubacterium eligens ATCC 27750
The biosynthesis of ubiquinol starts the interaction of 4-hydroxybenzoic acid interacting with an octaprenyl diphosphate. The former compound comes from the chorismate interacting with a chorismate lyase resulting in the release of a pyruvic acid and a 4-hydroxybenzoic acid. On the other hand, the latter compound, octaprenyl diphosphate is the result of a farnesyl pyrophosphate interacting with an isopentenyl pyrophosphate through an octaprenyl diphosphate synthase resulting in the release of a pyrophosphate and an octaprenyl diphosphate.
The 4-hydroxybenzoic acid interacts with octaprenyl diphosphate through a 4-hydroxybenzoate octaprenyltransferase resulting in the release of a pyrophosphate and a 3-octaprenyl-4-hydroxybenzoate. The latter compound then interacts with a hydrogen ion through a 3-octaprenyl-4-hydroxybenzoate carboxy-lyase resulting in the release of a carbon dioxide and a 2-octaprenylphenol. The latter compound interacts with an oxygen molecule and a hydrogen ion through a NADPH driven 2-octaprenylphenol hydroxylase resulting in a NADP, a water molecule and a 2-octaprenyl-6-hydroxyphenol.
The 2-octaprenyl-6-hydroxyphenol interacts with an S-adenosylmethionine through a bifunctional 3-demethylubiquinone-8 3-O-methyltransferase and 2-octaprenyl-6-hydroxyphenol methylase resulting in the release of a hydrogen ion, an s-adenosylhomocysteine and a 2-methoxy-6-(all-trans-octaprenyl)phenol. The latter compound then interacts with an oxygen molecule and a hydrogen ion through a NADPH driven 2-octaprenyl-6-methoxyphenol hydroxylase resulting in a NADP, a water molecule and a 2-methoxy-6-all trans-octaprenyl-2-methoxy-1,4-benzoquinol.
The latter compound interacts with a S-adenosylmethionine through a bifunctional 2-octaprenyl-6-methoxy-1,4-benzoquinone methylase and S-adenosylmethionine:2-DMK methyltransferase resulting in a s-adenosylhomocysteine, a hydrogen ion and a 6-methoxy-3-methyl-2-all-trans-octaprenyl-1,4-benzoquinol. The 6-methoxy-3-methyl-2-all-trans-octaprenyl-1,4-benzoquinol. interacts with a reduced acceptor, an oxygen molecule through a 2-octaprenyl-3-methyl-6-methoxy-1,4-benzoquinone hydroxylase resulting in the release of a water molecule, an oxidized electron acceptor and a 3-demethylubiquinol-8. The latter compound then interacts with a S-adenosylmethionine through a bifunctional 3-demethylubiquinone-8 3-O-methyltransferase and 2-octaprenyl-6-hydroxyphenol methylase resulting in a hydrogen ion, a S-adenosylhomocysteine and a ubiquinol 8.
|
Metabolite
Metabolic
|
|
|
SMP0452220![]() |
S-Adenosyl-L-Methionine BiosynthesisSuccinatimonas hippei YIT 12066
S-adenosyl-L-methionine biosynthesis(SAM) is synthesized in the cytosol of the cell from L-methionine and ATP. This reaction is catalyzed by methionine adenosyltransferase. L methione is taken up from the environment through a complex reaction coupled transport and then proceeds too synthesize the s adenosylmethionine through a adenosylmethionine synthase. S-adenosylmethionine then interacts with a hydrogen ion through an adenosylmethionine decarboxylase resulting in a carbon dioxide and a S-adenosyl 3-methioninamine. This compound interacts with a putrescine through a spermidine synthase resulting in a spermidine, a hydrogen ion and a S-methyl-5'-thioadenosine. The latter compound is degraded by interacting with a water molecule through a 5' methylthioadenosine nucleosidase resulting in an adenine and a S-methylthioribose which is then release into the environment
|
Metabolite
Metabolic
|
|
|
SMP0452216![]() |
Glutathione MetabolismSuccinatimonas hippei YIT 12066
The biosynthesis of glutathione starts with the introduction of L-glutamic acid through either a glutamate:sodium symporter, glutamate / aspartate : H+ symporter GltP or a
glutamate / aspartate ABC transporter. Once in the cytoplasm, L-glutamice acid reacts with L-cysteine through an ATP glutamate-cysteine ligase resulting in gamma-glutamylcysteine. This compound reacts which Glycine through an ATP driven glutathione synthetase thus catabolizing Glutathione.
This compound is metabolized through a spontaneous reaction with an oxidized glutaredoxin resulting in a reduced glutaredoxin and an oxidized glutathione. This compound is reduced by a NADPH glutathione reductase resulting in a glutathione.
Glutathione can then be degraded into various different glutathione containing compounds by reacting with a napthalene or Bromobenzene-2,3-oxide through a glutathione S-transferase.
|
Metabolite
Metabolic
|
|
|
SMP0347912 |
Cardiolipin Biosynthesis CL(8:0/a-13:0/18:2(9Z,11Z)/i-22:0)Homo sapiens
Cardiolipin (CL) is an important component of the inner mitochondrial membrane where it constitutes about 20% of the total lipid composition. It is essential for the optimal function of numerous enzymes that are involved in mitochondrial energy metabolism (Wikipedia). Cardiolipin biosynthesis occurs mainly in the mitochondria, but there also exists an alternative synthesis route for CDP-diacylglycerol that takes place in the endoplasmic reticulum. This second route may supplement this pathway. All membrane-localized enzymes are coloured dark green in the image. First, dihydroxyacetone phosphate (or glycerone phosphate) from glycolysis is used by the cytosolic enzyme glycerol-3-phosphate dehydrogenase [NAD(+)] to synthesize sn-glycerol 3-phosphate. Second, the mitochondrial outer membrane enzyme glycerol-3-phosphate acyltransferase esterifies an acyl-group to the sn-1 position of sn-glycerol 3-phosphate to form 1-acyl-sn-glycerol 3-phosphate (lysophosphatidic acid or LPA). Third, the enzyme 1-acyl-sn-glycerol-3-phosphate acyltransferase converts LPA into phosphatidic acid (PA or 1,2-diacyl-sn-glycerol 3-phosphate) by esterifying an acyl-group to the sn-2 position of the glycerol backbone. PA is then transferred to the inner mitochondrial membrane to continue cardiolipin synthesis. Fourth, magnesium-dependent phosphatidate cytidylyltransferase catalyzes the conversion of PA into CDP-diacylglycerol. Fifth, CDP-diacylglycerol--glycerol-3-phosphate 3-phosphatidyltransferase synthesizes phosphatidylglycerophosphate (PGP). Sixth, phosphatidylglycerophosphatase and protein-tyrosine phosphatase dephosphorylates PGP to form phosphatidylglycerol (PG). Last, cardiolipin synthase catalyzes the synthesis of cardiolipin by transferring a phosphatidyl group from a second CDP-diacylglycerol to PG. It requires a divalent metal cation cofactor.
|
Metabolite
Metabolic
|
|
Showing 372441 -
372450 of 372978 pathways