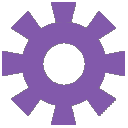
Browsing Pathways
Showing 372511 -
372520 of 605359 pathways
PathBank ID | Pathway Name and Description | Pathway Class | Chemical Compounds | Proteins |
---|---|---|---|---|
SMP0453959![]() |
Secondary Metabolites: Histidine BiosynthesisFilifactor alocis ATCC 35896
Histidine biosynthesis starts with a product of PRPP biosynthesis pathway, phosphoribosyl pyrophosphate which interacts with a hydrogen ion through an ATP phosphoribosyltransferase resulting in an pyrophosphate and a phosphoribosyl-ATP. The phosphoribosyl-ATP interacts with water through a phosphoribosyl-AMP cyclohydrolase / phosphoribosyl-ATP pyrophosphatase resulting in the release of pyrophosphate, hydrogen ion and a phosphoribosyl-AMP. The same enzyme proceeds to interact with phosphoribosyl-AMP and water resulting in a 1-(5'-Phosphoribosyl)-5-amino-4-imidazolecarboxamide. The product is then isomerized by a N-(5'-phospho-L-ribosyl-formimino)-5-amino-1-(5'-phosphoribosyl)-4-imidazolecarboxamide isomerase resulting in a PhosphoribosylformiminoAICAR-phosphate, which reacts with L-glutamine through an imidazole glycerol phosphate synthase resulting in a L-glutamic acid, hydrogen ion, 5-aminoimidazole-4-carboxamide and a D-erythro-imidazole-glycerol-phosphate. D-erythro-imidazole-glycerol-phosphate reacts with a imidazoleglycerol-phosphate dehydratase / histidinol-phosphatase, dehydrating the compound and resulting in a imidazole acetol-phosphate. Next, imidazole acetol-phosphate reacts with L-glutamic acid through a histidinol-phosphate aminotransferase, releasing oxoglutaric acid and L-histidinol-phosphate. The latter compound interacts with water and a imidazoleglycerol-phosphate dehydratase / histidinol-phosphatase resulting in L-histidinol and phosphate. L-histidinol interacts with a NAD-driven histidinol dehydrogenase resulting in a Histidinal. Histidinal in turn reacts with water in a NAD driven histidinal dehydrogenase resulting in L-Histidine.
L-Histidine then represses ATP phosphoribosyltransferase, regulation its own biosynthesis.
|
Metabolite
Metabolic
|
|
|
SMP0453965![]() |
Secondary Metabolites: Shikimate PathwayShigella flexneri 2002017
The biosynthesis of shikimate starts with D-Erythrose-4-phosphate getting transformed into 3-deoxy-D-arabino-heptulosonate-7-phosphate through a phospho-2-dehydro-3-deoxyheptonate aldolase. This is followed by a 3-dehydroquinate synthase converting the 3-deoxy-D-arabino-heptulosonate-7-phosphate into a 3-dehydroquinate which in turn is conveted to 3-dehydroshikimate through a 3-dehydroquinate dehydratase. A this point 3-dehydroshikimate can be turned into Shikimic acid through 2 different reactions involving an NADPH driven Quinate/shikimate dehydrogenase or a NADPH driven shikimate dehydrogenase 2.
Shikimate can also be transported through a shikimate:H+ symporter.
|
Metabolite
Metabolic
|
||
SMP0453953![]() |
beta-Alanine MetabolismMegamonas funiformis YIT 11815
Beta-Alanine metabolism starts as a product of aspartate metabolism. Aspartate is decarboxylated by aspartate 1-decarboxylase, releasing carbon dioxide and beta-alanine. Beta-Alanine is then metabolized through a pantothenate synthease resulting in pantothenic acid. Pantothenic acid then undergoes phosphorylation through an ATP-driven pantothenate kinase, resulting in D-4-phosphopantothenate. Pantothenate, vitamin B5, is a precursor for synthesis of 4'-phosphopantetheine moiety of coenzyme A and acyl carrier protein. Plants and microorganisms can synthesize pantothenate de novo, but animals must obtain it from diet. Enzymes of beta-alanine metabolism are targets for anti-microbial drugs.
|
Metabolite
Metabolic
|
||
SMP0351500 |
Cardiolipin Biosynthesis CL(a-13:0/18:2(9Z,11Z)/a-13:0/18:2(9Z,11Z))[rac]Homo sapiens
Cardiolipin (CL) is an important component of the inner mitochondrial membrane where it constitutes about 20% of the total lipid composition. It is essential for the optimal function of numerous enzymes that are involved in mitochondrial energy metabolism (Wikipedia). Cardiolipin biosynthesis occurs mainly in the mitochondria, but there also exists an alternative synthesis route for CDP-diacylglycerol that takes place in the endoplasmic reticulum. This second route may supplement this pathway. All membrane-localized enzymes are coloured dark green in the image. First, dihydroxyacetone phosphate (or glycerone phosphate) from glycolysis is used by the cytosolic enzyme glycerol-3-phosphate dehydrogenase [NAD(+)] to synthesize sn-glycerol 3-phosphate. Second, the mitochondrial outer membrane enzyme glycerol-3-phosphate acyltransferase esterifies an acyl-group to the sn-1 position of sn-glycerol 3-phosphate to form 1-acyl-sn-glycerol 3-phosphate (lysophosphatidic acid or LPA). Third, the enzyme 1-acyl-sn-glycerol-3-phosphate acyltransferase converts LPA into phosphatidic acid (PA or 1,2-diacyl-sn-glycerol 3-phosphate) by esterifying an acyl-group to the sn-2 position of the glycerol backbone. PA is then transferred to the inner mitochondrial membrane to continue cardiolipin synthesis. Fourth, magnesium-dependent phosphatidate cytidylyltransferase catalyzes the conversion of PA into CDP-diacylglycerol. Fifth, CDP-diacylglycerol--glycerol-3-phosphate 3-phosphatidyltransferase synthesizes phosphatidylglycerophosphate (PGP). Sixth, phosphatidylglycerophosphatase and protein-tyrosine phosphatase dephosphorylates PGP to form phosphatidylglycerol (PG). Last, cardiolipin synthase catalyzes the synthesis of cardiolipin by transferring a phosphatidyl group from a second CDP-diacylglycerol to PG. It requires a divalent metal cation cofactor.
|
Metabolite
Metabolic
|
|
|
SMP0453955![]() |
Secondary Metabolites: Ubiquinol BiosynthesisHaemophilus influenzae R2846
The biosynthesis of ubiquinol starts the interaction of 4-hydroxybenzoic acid interacting with an octaprenyl diphosphate. The former compound comes from the chorismate interacting with a chorismate lyase resulting in the release of a pyruvic acid and a 4-hydroxybenzoic acid. On the other hand, the latter compound, octaprenyl diphosphate is the result of a farnesyl pyrophosphate interacting with an isopentenyl pyrophosphate through an octaprenyl diphosphate synthase resulting in the release of a pyrophosphate and an octaprenyl diphosphate.
The 4-hydroxybenzoic acid interacts with octaprenyl diphosphate through a 4-hydroxybenzoate octaprenyltransferase resulting in the release of a pyrophosphate and a 3-octaprenyl-4-hydroxybenzoate. The latter compound then interacts with a hydrogen ion through a 3-octaprenyl-4-hydroxybenzoate carboxy-lyase resulting in the release of a carbon dioxide and a 2-octaprenylphenol. The latter compound interacts with an oxygen molecule and a hydrogen ion through a NADPH driven 2-octaprenylphenol hydroxylase resulting in a NADP, a water molecule and a 2-octaprenyl-6-hydroxyphenol.
The 2-octaprenyl-6-hydroxyphenol interacts with an S-adenosylmethionine through a bifunctional 3-demethylubiquinone-8 3-O-methyltransferase and 2-octaprenyl-6-hydroxyphenol methylase resulting in the release of a hydrogen ion, an s-adenosylhomocysteine and a 2-methoxy-6-(all-trans-octaprenyl)phenol. The latter compound then interacts with an oxygen molecule and a hydrogen ion through a NADPH driven 2-octaprenyl-6-methoxyphenol hydroxylase resulting in a NADP, a water molecule and a 2-methoxy-6-all trans-octaprenyl-2-methoxy-1,4-benzoquinol.
The latter compound interacts with a S-adenosylmethionine through a bifunctional 2-octaprenyl-6-methoxy-1,4-benzoquinone methylase and S-adenosylmethionine:2-DMK methyltransferase resulting in a s-adenosylhomocysteine, a hydrogen ion and a 6-methoxy-3-methyl-2-all-trans-octaprenyl-1,4-benzoquinol. The 6-methoxy-3-methyl-2-all-trans-octaprenyl-1,4-benzoquinol. interacts with a reduced acceptor, an oxygen molecule through a 2-octaprenyl-3-methyl-6-methoxy-1,4-benzoquinone hydroxylase resulting in the release of a water molecule, an oxidized electron acceptor and a 3-demethylubiquinol-8. The latter compound then interacts with a S-adenosylmethionine through a bifunctional 3-demethylubiquinone-8 3-O-methyltransferase and 2-octaprenyl-6-hydroxyphenol methylase resulting in a hydrogen ion, a S-adenosylhomocysteine and a ubiquinol 8.
|
Metabolite
Metabolic
|
|
|
SMP0454060![]() |
Amino Sugar and Nucleotide Sugar Metabolism IIIDialister succinatiphilus YIT 11850
The synthesis of amino sugars and nucleotide sugars starts with the phosphorylation of N-Acetylmuramic acid (MurNac) through its transport from the periplasmic space to the cytoplasm. Once in the cytoplasm, MurNac and water undergo a reversible reaction catalyzed by N-acetylmuramic acid 6-phosphate etherase, producing a D-lactic acid and N-Acetyl-D-Glucosamine 6-phosphate. This latter compound can also be introduced into the cytoplasm through a phosphorylating PTS permase in the inner membrane that allows for the transport of N-Acetyl-D-glucosamine from the periplasmic space. N-Acetyl-D-Glucosamine 6-phosphate can also be obtained from chitin dependent reactions. Chitin is hydrated through a bifunctional chitinase to produce chitobiose. This in turn gets hydrated by a beta-hexosaminidase to produce N-acetyl-D-glucosamine. The latter undergoes an atp dependent phosphorylation leading to the production of N-Acetyl-D-Glucosamine 6-phosphate. N-Acetyl-D-Glucosamine 6-phosphate is then be deacetylated in order to produce Glucosamine 6-phosphate through a N-acetylglucosamine-6-phosphate deacetylase. This compound can either be isomerized or deaminated into Beta-D-fructofuranose 6-phosphate through a glucosamine-fructose-6-phosphate aminotransferase and a glucosamine-6-phosphate deaminase respectively.
Glucosamine 6-phosphate undergoes a reversible reaction to glucosamine 1 phosphate through a phosphoglucosamine mutase. This compound is then acetylated through a bifunctional protein glmU to produce a N-Acetyl glucosamine 1-phosphate.
N-Acetyl glucosamine 1-phosphate enters the nucleotide sugar synthesis by reacting with UTP and hydrogen ion through a bifunctional protein glmU releasing pyrophosphate and a Uridine diphosphate-N-acetylglucosamine.This compound can either be isomerized into a UDP-N-acetyl-D-mannosamine or undergo a reaction with phosphoenolpyruvic acid through UDP-N-acetylglucosamine 1-carboxyvinyltransferase releasing a phosphate and a UDP-N-Acetyl-alpha-D-glucosamine-enolpyruvate.
UDP-N-acetyl-D-mannosamine undergoes a NAD dependent dehydrogenation through a UDP-N-acetyl-D-mannosamine dehydrogenase, releasing NADH, a hydrogen ion and a UDP-N-Acetyl-alpha-D-mannosaminuronate, This compound is then used in the production of enterobacterial common antigens.
UDP-N-Acetyl-alpha-D-glucosamine-enolpyruvate is reduced through a NADPH dependent UDP-N-acetylenolpyruvoylglucosamine reductase, releasing a NADP and a UDP-N-acetyl-alpha-D-muramate. This compound is involved in the D-glutamine and D-glutamate metabolism.
|
Metabolite
Metabolic
|
|
|
SMP0454069![]() |
GTP Degradation and Molybdenum Cofactor BiosynthesisProteus mirabilis HI4320
GTP, produced in the nucleotide de novo biosyntheis pathway, interacts with a water molecule through a GTP cyclohydrolase resulting in a formate, hydrogen ion and a 7,8-dihydroneopterin 3'-triphosphate. The latter compound interacts with a water molecule through a dihydroneopterin triphosphate pyrophosphohydrolase resulting in the release of a pyrophosphate, a hydrogen ion and a 7,8-dihydroneopterin 3'-phosphate. The latter compound interacts with water spontaneously resulting in the release of a phosphate and a 7,8 dihydroneopterin. The latter compound interacts with a dihydroneopterin aldolase resulting in the release of a glycolaldehyde and a 6-hydroxymethyl-7,8-dihydropterin. This compound then is then diphosphorylated by reacting with a ATP driven 6-hydroxymethyl-7,8-dihydropterin pyrophosphokinase resulting in the release of a hydrogen ion, an AMP and 6-hydroxymethyl-7,8-dihydropterin diphosphate.
GTP interacts with a cyclic pyranopterin monophosphate synthase resulting in the release of a diphosphate and a cyclic pyranopterin phosphate. The latter compound interacts with a thiocarboxylated small subunit of molybdopterin synthase (a protein) and a water molecule through a molybdopterin synthase resulting in the release of 4 hydrogen ions, 2 small subunits of molybdopterin synthase and a molybdopterin. The molybdopterin interacts with an ATP and a hydrogen ion through a molybdopterin adenylyltransferase resulting in the release of a diphosphate and a molybdopterin adenine dinucleotide. The latter compound is then metabolized by a hydrogen ion and a molybdate through a molybdopterin molybdenumtransferase resulting in the release of an AMP, a water molecule and a molybdopterin cofactor.
The molybdopterin cofactor can procede to the guanylyl molybdenum cofactor biosynthesis pathway or it can be metabolized into a cytidylyl molybdenum cofactor by interacting with a CTP and a hydrogen ion through a molybdenym cofactor cytidylyltransferase resulting in the release of a pyrophosphate and a cytidyllyl molybdenum cofactor
|
Metabolite
Metabolic
|
|
|
SMP0454055![]() |
Adenosylcobalamin Salvage from CobinamidePorphyromonas gingivalis W83
Cobinamide is incorporated from the extracellular space through a transport system into the cytosol. Once inside the cytosol, cobinamide interacts with ATP through a cobinamide adenosyl transferase resulting in the release of a triphosphate and an adenosylcobinamide. The latter compound is then phosphorylated through an ATP-dependent cobinamide kinase resulting in the release of ADP, a hydrogen ion and adenosyl-cobinamide phosphate. This last compound then interacts with GTP and a hydrogen ion through a cobinamide-P guanylyltransferase resulting in the release of a pyrophosphate and an adenosylcobinamide-GDP.
A dimethylbenzimidazole interacts with a nicotinate D-ribonucleotide through a nicotinate-nucleotide dimethylbenzumidazole phosphoribosyltransferase resulting in the release of a nicotinate, a hydrogen ion and an alpha-ribazole 5' phosphate.
The adenosylcobinamide-GDP and the alpha-ribazole 5' phosphate interact together through a cobalamin 5' phosphate synthase resulting in the release of a hydrogen ion, a GMP and Adenosylcobalamin 5'-phosphate. The latter compound then interacts with a water molecule through an adenosylcbalamin 5' phosphate phosphatase resulting in the release of a phosphate and a coenzyme B12.
Likewise a cobalamin molecule can interact with ATP through a cobalamin adenosyltransferase resulting in the release of a triphosphate and a coenzyme B12
|
Metabolite
Metabolic
|
|
|
SMP0352288 |
Cardiolipin Biosynthesis CL(a-13:0/18:2(9Z,11Z)/a-17:0/i-24:0)[rac]Homo sapiens
Cardiolipin (CL) is an important component of the inner mitochondrial membrane where it constitutes about 20% of the total lipid composition. It is essential for the optimal function of numerous enzymes that are involved in mitochondrial energy metabolism (Wikipedia). Cardiolipin biosynthesis occurs mainly in the mitochondria, but there also exists an alternative synthesis route for CDP-diacylglycerol that takes place in the endoplasmic reticulum. This second route may supplement this pathway. All membrane-localized enzymes are coloured dark green in the image. First, dihydroxyacetone phosphate (or glycerone phosphate) from glycolysis is used by the cytosolic enzyme glycerol-3-phosphate dehydrogenase [NAD(+)] to synthesize sn-glycerol 3-phosphate. Second, the mitochondrial outer membrane enzyme glycerol-3-phosphate acyltransferase esterifies an acyl-group to the sn-1 position of sn-glycerol 3-phosphate to form 1-acyl-sn-glycerol 3-phosphate (lysophosphatidic acid or LPA). Third, the enzyme 1-acyl-sn-glycerol-3-phosphate acyltransferase converts LPA into phosphatidic acid (PA or 1,2-diacyl-sn-glycerol 3-phosphate) by esterifying an acyl-group to the sn-2 position of the glycerol backbone. PA is then transferred to the inner mitochondrial membrane to continue cardiolipin synthesis. Fourth, magnesium-dependent phosphatidate cytidylyltransferase catalyzes the conversion of PA into CDP-diacylglycerol. Fifth, CDP-diacylglycerol--glycerol-3-phosphate 3-phosphatidyltransferase synthesizes phosphatidylglycerophosphate (PGP). Sixth, phosphatidylglycerophosphatase and protein-tyrosine phosphatase dephosphorylates PGP to form phosphatidylglycerol (PG). Last, cardiolipin synthase catalyzes the synthesis of cardiolipin by transferring a phosphatidyl group from a second CDP-diacylglycerol to PG. It requires a divalent metal cation cofactor.
|
Metabolite
Metabolic
|
|
|
SMP0352286 |
Cardiolipin Biosynthesis CL(a-13:0/18:2(9Z,11Z)/a-17:0/i-21:0)[rac]Homo sapiens
Cardiolipin (CL) is an important component of the inner mitochondrial membrane where it constitutes about 20% of the total lipid composition. It is essential for the optimal function of numerous enzymes that are involved in mitochondrial energy metabolism (Wikipedia). Cardiolipin biosynthesis occurs mainly in the mitochondria, but there also exists an alternative synthesis route for CDP-diacylglycerol that takes place in the endoplasmic reticulum. This second route may supplement this pathway. All membrane-localized enzymes are coloured dark green in the image. First, dihydroxyacetone phosphate (or glycerone phosphate) from glycolysis is used by the cytosolic enzyme glycerol-3-phosphate dehydrogenase [NAD(+)] to synthesize sn-glycerol 3-phosphate. Second, the mitochondrial outer membrane enzyme glycerol-3-phosphate acyltransferase esterifies an acyl-group to the sn-1 position of sn-glycerol 3-phosphate to form 1-acyl-sn-glycerol 3-phosphate (lysophosphatidic acid or LPA). Third, the enzyme 1-acyl-sn-glycerol-3-phosphate acyltransferase converts LPA into phosphatidic acid (PA or 1,2-diacyl-sn-glycerol 3-phosphate) by esterifying an acyl-group to the sn-2 position of the glycerol backbone. PA is then transferred to the inner mitochondrial membrane to continue cardiolipin synthesis. Fourth, magnesium-dependent phosphatidate cytidylyltransferase catalyzes the conversion of PA into CDP-diacylglycerol. Fifth, CDP-diacylglycerol--glycerol-3-phosphate 3-phosphatidyltransferase synthesizes phosphatidylglycerophosphate (PGP). Sixth, phosphatidylglycerophosphatase and protein-tyrosine phosphatase dephosphorylates PGP to form phosphatidylglycerol (PG). Last, cardiolipin synthase catalyzes the synthesis of cardiolipin by transferring a phosphatidyl group from a second CDP-diacylglycerol to PG. It requires a divalent metal cation cofactor.
|
Metabolite
Metabolic
|
|
Showing 372511 -
372520 of 372822 pathways