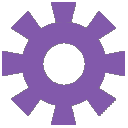
Browsing Pathways
Showing 373291 -
373300 of 605359 pathways
PathBank ID | Pathway Name and Description | Pathway Class | Chemical Compounds | Proteins |
---|---|---|---|---|
SMP0452865![]() |
GTP Degradation and Molybdenum Cofactor BiosynthesisBuchnera aphidicola str. 5A (Acyrthosiphon pisum)
GTP, produced in the nucleotide de novo biosyntheis pathway, interacts with a water molecule through a GTP cyclohydrolase resulting in a formate, hydrogen ion and a 7,8-dihydroneopterin 3'-triphosphate. The latter compound interacts with a water molecule through a dihydroneopterin triphosphate pyrophosphohydrolase resulting in the release of a pyrophosphate, a hydrogen ion and a 7,8-dihydroneopterin 3'-phosphate. The latter compound interacts with water spontaneously resulting in the release of a phosphate and a 7,8 dihydroneopterin. The latter compound interacts with a dihydroneopterin aldolase resulting in the release of a glycolaldehyde and a 6-hydroxymethyl-7,8-dihydropterin. This compound then is then diphosphorylated by reacting with a ATP driven 6-hydroxymethyl-7,8-dihydropterin pyrophosphokinase resulting in the release of a hydrogen ion, an AMP and 6-hydroxymethyl-7,8-dihydropterin diphosphate.
GTP interacts with a cyclic pyranopterin monophosphate synthase resulting in the release of a diphosphate and a cyclic pyranopterin phosphate. The latter compound interacts with a thiocarboxylated small subunit of molybdopterin synthase (a protein) and a water molecule through a molybdopterin synthase resulting in the release of 4 hydrogen ions, 2 small subunits of molybdopterin synthase and a molybdopterin. The molybdopterin interacts with an ATP and a hydrogen ion through a molybdopterin adenylyltransferase resulting in the release of a diphosphate and a molybdopterin adenine dinucleotide. The latter compound is then metabolized by a hydrogen ion and a molybdate through a molybdopterin molybdenumtransferase resulting in the release of an AMP, a water molecule and a molybdopterin cofactor.
The molybdopterin cofactor can procede to the guanylyl molybdenum cofactor biosynthesis pathway or it can be metabolized into a cytidylyl molybdenum cofactor by interacting with a CTP and a hydrogen ion through a molybdenym cofactor cytidylyltransferase resulting in the release of a pyrophosphate and a cytidyllyl molybdenum cofactor
|
Metabolite
Metabolic
|
|
|
SMP0349830 |
Cardiolipin Biosynthesis CL(a-13:0/a-13:0/19:0/i-24:0)Homo sapiens
Cardiolipin (CL) is an important component of the inner mitochondrial membrane where it constitutes about 20% of the total lipid composition. It is essential for the optimal function of numerous enzymes that are involved in mitochondrial energy metabolism (Wikipedia). Cardiolipin biosynthesis occurs mainly in the mitochondria, but there also exists an alternative synthesis route for CDP-diacylglycerol that takes place in the endoplasmic reticulum. This second route may supplement this pathway. All membrane-localized enzymes are coloured dark green in the image. First, dihydroxyacetone phosphate (or glycerone phosphate) from glycolysis is used by the cytosolic enzyme glycerol-3-phosphate dehydrogenase [NAD(+)] to synthesize sn-glycerol 3-phosphate. Second, the mitochondrial outer membrane enzyme glycerol-3-phosphate acyltransferase esterifies an acyl-group to the sn-1 position of sn-glycerol 3-phosphate to form 1-acyl-sn-glycerol 3-phosphate (lysophosphatidic acid or LPA). Third, the enzyme 1-acyl-sn-glycerol-3-phosphate acyltransferase converts LPA into phosphatidic acid (PA or 1,2-diacyl-sn-glycerol 3-phosphate) by esterifying an acyl-group to the sn-2 position of the glycerol backbone. PA is then transferred to the inner mitochondrial membrane to continue cardiolipin synthesis. Fourth, magnesium-dependent phosphatidate cytidylyltransferase catalyzes the conversion of PA into CDP-diacylglycerol. Fifth, CDP-diacylglycerol--glycerol-3-phosphate 3-phosphatidyltransferase synthesizes phosphatidylglycerophosphate (PGP). Sixth, phosphatidylglycerophosphatase and protein-tyrosine phosphatase dephosphorylates PGP to form phosphatidylglycerol (PG). Last, cardiolipin synthase catalyzes the synthesis of cardiolipin by transferring a phosphatidyl group from a second CDP-diacylglycerol to PG. It requires a divalent metal cation cofactor.
|
Metabolite
Metabolic
|
|
|
SMP0452966![]() |
Secondary Metabolites: Histidine BiosynthesisCampylobacter curvus 525.92
Histidine biosynthesis starts with a product of PRPP biosynthesis pathway, phosphoribosyl pyrophosphate which interacts with a hydrogen ion through an ATP phosphoribosyltransferase resulting in an pyrophosphate and a phosphoribosyl-ATP. The phosphoribosyl-ATP interacts with water through a phosphoribosyl-AMP cyclohydrolase / phosphoribosyl-ATP pyrophosphatase resulting in the release of pyrophosphate, hydrogen ion and a phosphoribosyl-AMP. The same enzyme proceeds to interact with phosphoribosyl-AMP and water resulting in a 1-(5'-Phosphoribosyl)-5-amino-4-imidazolecarboxamide. The product is then isomerized by a N-(5'-phospho-L-ribosyl-formimino)-5-amino-1-(5'-phosphoribosyl)-4-imidazolecarboxamide isomerase resulting in a PhosphoribosylformiminoAICAR-phosphate, which reacts with L-glutamine through an imidazole glycerol phosphate synthase resulting in a L-glutamic acid, hydrogen ion, 5-aminoimidazole-4-carboxamide and a D-erythro-imidazole-glycerol-phosphate. D-erythro-imidazole-glycerol-phosphate reacts with a imidazoleglycerol-phosphate dehydratase / histidinol-phosphatase, dehydrating the compound and resulting in a imidazole acetol-phosphate. Next, imidazole acetol-phosphate reacts with L-glutamic acid through a histidinol-phosphate aminotransferase, releasing oxoglutaric acid and L-histidinol-phosphate. The latter compound interacts with water and a imidazoleglycerol-phosphate dehydratase / histidinol-phosphatase resulting in L-histidinol and phosphate. L-histidinol interacts with a NAD-driven histidinol dehydrogenase resulting in a Histidinal. Histidinal in turn reacts with water in a NAD driven histidinal dehydrogenase resulting in L-Histidine.
L-Histidine then represses ATP phosphoribosyltransferase, regulation its own biosynthesis.
|
Metabolite
Metabolic
|
|
|
SMP0452962![]() |
Secondary Metabolites: Ubiquinol BiosynthesisCampylobacter concisus 13826
The biosynthesis of ubiquinol starts the interaction of 4-hydroxybenzoic acid interacting with an octaprenyl diphosphate. The former compound comes from the chorismate interacting with a chorismate lyase resulting in the release of a pyruvic acid and a 4-hydroxybenzoic acid. On the other hand, the latter compound, octaprenyl diphosphate is the result of a farnesyl pyrophosphate interacting with an isopentenyl pyrophosphate through an octaprenyl diphosphate synthase resulting in the release of a pyrophosphate and an octaprenyl diphosphate.
The 4-hydroxybenzoic acid interacts with octaprenyl diphosphate through a 4-hydroxybenzoate octaprenyltransferase resulting in the release of a pyrophosphate and a 3-octaprenyl-4-hydroxybenzoate. The latter compound then interacts with a hydrogen ion through a 3-octaprenyl-4-hydroxybenzoate carboxy-lyase resulting in the release of a carbon dioxide and a 2-octaprenylphenol. The latter compound interacts with an oxygen molecule and a hydrogen ion through a NADPH driven 2-octaprenylphenol hydroxylase resulting in a NADP, a water molecule and a 2-octaprenyl-6-hydroxyphenol.
The 2-octaprenyl-6-hydroxyphenol interacts with an S-adenosylmethionine through a bifunctional 3-demethylubiquinone-8 3-O-methyltransferase and 2-octaprenyl-6-hydroxyphenol methylase resulting in the release of a hydrogen ion, an s-adenosylhomocysteine and a 2-methoxy-6-(all-trans-octaprenyl)phenol. The latter compound then interacts with an oxygen molecule and a hydrogen ion through a NADPH driven 2-octaprenyl-6-methoxyphenol hydroxylase resulting in a NADP, a water molecule and a 2-methoxy-6-all trans-octaprenyl-2-methoxy-1,4-benzoquinol.
The latter compound interacts with a S-adenosylmethionine through a bifunctional 2-octaprenyl-6-methoxy-1,4-benzoquinone methylase and S-adenosylmethionine:2-DMK methyltransferase resulting in a s-adenosylhomocysteine, a hydrogen ion and a 6-methoxy-3-methyl-2-all-trans-octaprenyl-1,4-benzoquinol. The 6-methoxy-3-methyl-2-all-trans-octaprenyl-1,4-benzoquinol. interacts with a reduced acceptor, an oxygen molecule through a 2-octaprenyl-3-methyl-6-methoxy-1,4-benzoquinone hydroxylase resulting in the release of a water molecule, an oxidized electron acceptor and a 3-demethylubiquinol-8. The latter compound then interacts with a S-adenosylmethionine through a bifunctional 3-demethylubiquinone-8 3-O-methyltransferase and 2-octaprenyl-6-hydroxyphenol methylase resulting in a hydrogen ion, a S-adenosylhomocysteine and a ubiquinol 8.
|
Metabolite
Metabolic
|
|
|
SMP0349818 |
Cardiolipin Biosynthesis CL(a-13:0/a-13:0/19:0/i-19:0)Homo sapiens
Cardiolipin (CL) is an important component of the inner mitochondrial membrane where it constitutes about 20% of the total lipid composition. It is essential for the optimal function of numerous enzymes that are involved in mitochondrial energy metabolism (Wikipedia). Cardiolipin biosynthesis occurs mainly in the mitochondria, but there also exists an alternative synthesis route for CDP-diacylglycerol that takes place in the endoplasmic reticulum. This second route may supplement this pathway. All membrane-localized enzymes are coloured dark green in the image. First, dihydroxyacetone phosphate (or glycerone phosphate) from glycolysis is used by the cytosolic enzyme glycerol-3-phosphate dehydrogenase [NAD(+)] to synthesize sn-glycerol 3-phosphate. Second, the mitochondrial outer membrane enzyme glycerol-3-phosphate acyltransferase esterifies an acyl-group to the sn-1 position of sn-glycerol 3-phosphate to form 1-acyl-sn-glycerol 3-phosphate (lysophosphatidic acid or LPA). Third, the enzyme 1-acyl-sn-glycerol-3-phosphate acyltransferase converts LPA into phosphatidic acid (PA or 1,2-diacyl-sn-glycerol 3-phosphate) by esterifying an acyl-group to the sn-2 position of the glycerol backbone. PA is then transferred to the inner mitochondrial membrane to continue cardiolipin synthesis. Fourth, magnesium-dependent phosphatidate cytidylyltransferase catalyzes the conversion of PA into CDP-diacylglycerol. Fifth, CDP-diacylglycerol--glycerol-3-phosphate 3-phosphatidyltransferase synthesizes phosphatidylglycerophosphate (PGP). Sixth, phosphatidylglycerophosphatase and protein-tyrosine phosphatase dephosphorylates PGP to form phosphatidylglycerol (PG). Last, cardiolipin synthase catalyzes the synthesis of cardiolipin by transferring a phosphatidyl group from a second CDP-diacylglycerol to PG. It requires a divalent metal cation cofactor.
|
Metabolite
Metabolic
|
|
|
SMP0452958![]() |
Secondary Metabolites: Leucine BiosynthesisCampylobacter lari RM2100
Leucine biosynthesis involves a five-step conversion process starting with a 3-methyl-2-oxovaleric acid interacting with acetyl-CoA and a water molecule through a 2-isopropylmalate synthase resulting in Coenzyme A, hydrogen Ion and 2-isopropylmalic acid. The latter compound reacts with isopropylmalate isomerase which dehydrates the compound resulting in a Isopropylmaleate. This compound reacts with water through a isopropylmalate isomerase resulting in 3-isopropylmalate. This compound interacts with a NAD-driven D-malate / 3-isopropylmalate dehydrogenase results in 2-isopropyl-3-oxosuccinate. This compound interacts spontaneously with hydrogen resulting in the release of carbon dioxide and ketoleucine. Ketoleucine interacts in a reversible reaction with L-glutamic acid through a branched-chain amino-acid aminotransferase resulting in Oxoglutaric acid and L-leucine. 2-isopropylmalate synthase and terminal transaminase TyrB can both be suppressed by leucine. 2-keto-isovalerate and tyrosine can both inhibit the TyrB, which lead to absence of IlvE activity. Without IlvE activity, 2-ketoisocaproate could not convert to leucine since branched-chain amino-acid aminotransferase (IlvE) is the only enzyme to facilitate the reaction.
|
Metabolite
Metabolic
|
||
SMP0452978![]() |
Phospholipid BiosynthesisCampylobacter curvus 525.92
Phospholipids are membrane components in E. coli. The major phospholipids of E. coli are phosphatidylethanolamine, phosphatidylglycerol, and cardiolipin. All phospholipids contain sn-glycerol-3-phosphate esterified with fatty acids at the sn-1 and sn-2 positions. The reaction starts from a glycerone phosphate (dihydroxyacetone phosphate) produced in glycolysis. The glycerone phosphate is transformed into an sn-glycerol 3-phosphate (glycerol 3 phosphate) by NADPH-driven glycerol-3-phosphate dehydrogenase. sn-Glycerol 3-phosphate is transformed to a 1-acyl-sn-glycerol 3-phosphate (lysophosphatidic acid). This can be achieved by an sn-glycerol-3-phosphate acyltransferase that interacts either with a long-chain acyl-CoA or with an acyl-[acp]. The 1-acyl-sn-glycerol 3-phosphate is transformed into a 1,2-diacyl-sn-glycerol 3-phosphate (phosphatidic acid) through a 1-acylglycerol-3-phosphate O-acyltransferase. This compound is then converted into a CPD-diacylglycerol through a CTP phosphatidate cytididyltransferase. CPD-diacylglycerol can be transformed either into an L-1-phosphatidylserine or an L-1-phosphatidylglycerol-phosphate through a phosphatidylserine synthase or a phosphatidylglycerophosphate synthase, respectively. The L-1-phosphatidylserine transforms into L-1-phosphatidylethanolamine through a phosphatidylserine decarboxylase. On the other hand, L-1-phosphatidylglycerol-phosphate gets transformed into an L-1-phosphatidyl-glycerol through a phosphatidylglycerophosphatase. These 2 products combine to produce a cardiolipin and an ethanolamine. The L-1 phosphatidyl-glycerol can also interact with cardiolipin synthase resulting in a glycerol and a cardiolipin.
|
Metabolite
Metabolic
|
||
SMP0452973![]() |
Secondary Metabolites: Shikimate PathwayCampylobacter curvus 525.92
The biosynthesis of shikimate starts with D-Erythrose-4-phosphate getting transformed into 3-deoxy-D-arabino-heptulosonate-7-phosphate through a phospho-2-dehydro-3-deoxyheptonate aldolase. This is followed by a 3-dehydroquinate synthase converting the 3-deoxy-D-arabino-heptulosonate-7-phosphate into a 3-dehydroquinate which in turn is conveted to 3-dehydroshikimate through a 3-dehydroquinate dehydratase. A this point 3-dehydroshikimate can be turned into Shikimic acid through 2 different reactions involving an NADPH driven Quinate/shikimate dehydrogenase or a NADPH driven shikimate dehydrogenase 2.
Shikimate can also be transported through a shikimate:H+ symporter.
|
Metabolite
Metabolic
|
||
SMP0350176 |
Cardiolipin Biosynthesis CL(a-13:0/a-13:0/i-13:0/24:0)Homo sapiens
Cardiolipin (CL) is an important component of the inner mitochondrial membrane where it constitutes about 20% of the total lipid composition. It is essential for the optimal function of numerous enzymes that are involved in mitochondrial energy metabolism (Wikipedia). Cardiolipin biosynthesis occurs mainly in the mitochondria, but there also exists an alternative synthesis route for CDP-diacylglycerol that takes place in the endoplasmic reticulum. This second route may supplement this pathway. All membrane-localized enzymes are coloured dark green in the image. First, dihydroxyacetone phosphate (or glycerone phosphate) from glycolysis is used by the cytosolic enzyme glycerol-3-phosphate dehydrogenase [NAD(+)] to synthesize sn-glycerol 3-phosphate. Second, the mitochondrial outer membrane enzyme glycerol-3-phosphate acyltransferase esterifies an acyl-group to the sn-1 position of sn-glycerol 3-phosphate to form 1-acyl-sn-glycerol 3-phosphate (lysophosphatidic acid or LPA). Third, the enzyme 1-acyl-sn-glycerol-3-phosphate acyltransferase converts LPA into phosphatidic acid (PA or 1,2-diacyl-sn-glycerol 3-phosphate) by esterifying an acyl-group to the sn-2 position of the glycerol backbone. PA is then transferred to the inner mitochondrial membrane to continue cardiolipin synthesis. Fourth, magnesium-dependent phosphatidate cytidylyltransferase catalyzes the conversion of PA into CDP-diacylglycerol. Fifth, CDP-diacylglycerol--glycerol-3-phosphate 3-phosphatidyltransferase synthesizes phosphatidylglycerophosphate (PGP). Sixth, phosphatidylglycerophosphatase and protein-tyrosine phosphatase dephosphorylates PGP to form phosphatidylglycerol (PG). Last, cardiolipin synthase catalyzes the synthesis of cardiolipin by transferring a phosphatidyl group from a second CDP-diacylglycerol to PG. It requires a divalent metal cation cofactor.
|
Metabolite
Metabolic
|
|
|
SMP0453146![]() |
Secondary Metabolites: Histidine BiosynthesisEscherichia coli O157:H7 str. Sakai
Histidine biosynthesis starts with a product of PRPP biosynthesis pathway, phosphoribosyl pyrophosphate which interacts with a hydrogen ion through an ATP phosphoribosyltransferase resulting in an pyrophosphate and a phosphoribosyl-ATP. The phosphoribosyl-ATP interacts with water through a phosphoribosyl-AMP cyclohydrolase / phosphoribosyl-ATP pyrophosphatase resulting in the release of pyrophosphate, hydrogen ion and a phosphoribosyl-AMP. The same enzyme proceeds to interact with phosphoribosyl-AMP and water resulting in a 1-(5'-Phosphoribosyl)-5-amino-4-imidazolecarboxamide. The product is then isomerized by a N-(5'-phospho-L-ribosyl-formimino)-5-amino-1-(5'-phosphoribosyl)-4-imidazolecarboxamide isomerase resulting in a PhosphoribosylformiminoAICAR-phosphate, which reacts with L-glutamine through an imidazole glycerol phosphate synthase resulting in a L-glutamic acid, hydrogen ion, 5-aminoimidazole-4-carboxamide and a D-erythro-imidazole-glycerol-phosphate. D-erythro-imidazole-glycerol-phosphate reacts with a imidazoleglycerol-phosphate dehydratase / histidinol-phosphatase, dehydrating the compound and resulting in a imidazole acetol-phosphate. Next, imidazole acetol-phosphate reacts with L-glutamic acid through a histidinol-phosphate aminotransferase, releasing oxoglutaric acid and L-histidinol-phosphate. The latter compound interacts with water and a imidazoleglycerol-phosphate dehydratase / histidinol-phosphatase resulting in L-histidinol and phosphate. L-histidinol interacts with a NAD-driven histidinol dehydrogenase resulting in a Histidinal. Histidinal in turn reacts with water in a NAD driven histidinal dehydrogenase resulting in L-Histidine.
L-Histidine then represses ATP phosphoribosyltransferase, regulation its own biosynthesis.
|
Metabolite
Metabolic
|
|
Showing 373291 -
373300 of 376042 pathways