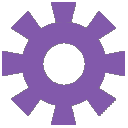
Browsing Pathways
Showing 375541 -
375550 of 605359 pathways
PathBank ID | Pathway Name and Description | Pathway Class | Chemical Compounds | Proteins |
---|---|---|---|---|
SMP0456873![]() |
GTP Degradation and Molybdenum Cofactor BiosynthesisHaemophilus haemolyticus M19501
GTP, produced in the nucleotide de novo biosyntheis pathway, interacts with a water molecule through a GTP cyclohydrolase resulting in a formate, hydrogen ion and a 7,8-dihydroneopterin 3'-triphosphate. The latter compound interacts with a water molecule through a dihydroneopterin triphosphate pyrophosphohydrolase resulting in the release of a pyrophosphate, a hydrogen ion and a 7,8-dihydroneopterin 3'-phosphate. The latter compound interacts with water spontaneously resulting in the release of a phosphate and a 7,8 dihydroneopterin. The latter compound interacts with a dihydroneopterin aldolase resulting in the release of a glycolaldehyde and a 6-hydroxymethyl-7,8-dihydropterin. This compound then is then diphosphorylated by reacting with a ATP driven 6-hydroxymethyl-7,8-dihydropterin pyrophosphokinase resulting in the release of a hydrogen ion, an AMP and 6-hydroxymethyl-7,8-dihydropterin diphosphate.
GTP interacts with a cyclic pyranopterin monophosphate synthase resulting in the release of a diphosphate and a cyclic pyranopterin phosphate. The latter compound interacts with a thiocarboxylated small subunit of molybdopterin synthase (a protein) and a water molecule through a molybdopterin synthase resulting in the release of 4 hydrogen ions, 2 small subunits of molybdopterin synthase and a molybdopterin. The molybdopterin interacts with an ATP and a hydrogen ion through a molybdopterin adenylyltransferase resulting in the release of a diphosphate and a molybdopterin adenine dinucleotide. The latter compound is then metabolized by a hydrogen ion and a molybdate through a molybdopterin molybdenumtransferase resulting in the release of an AMP, a water molecule and a molybdopterin cofactor.
The molybdopterin cofactor can procede to the guanylyl molybdenum cofactor biosynthesis pathway or it can be metabolized into a cytidylyl molybdenum cofactor by interacting with a CTP and a hydrogen ion through a molybdenym cofactor cytidylyltransferase resulting in the release of a pyrophosphate and a cytidyllyl molybdenum cofactor
|
Metabolite
Metabolic
|
|
|
SMP0456860![]() |
Secondary Metabolites: Glyoxylate CycleChlamydia trachomatis D/UW-3/CX
The glyoxylate cycle starts with the interaction of Acetyl-Coa with a water molecule and Oxalacetic acid interact through a Citrate synthase resulting in a release of a coenzyme a and citric acid. The citric acid gets dehydrated through a citrate hydro-lyase resulting in the release of a water molecule and cis-Aconitic acid. The cis-Aconitic acid is then hydrated in an reversible reaction through an aconitate hydratase resulting in an Isocitric acid. The isocitric acid then interacts in a reversible reaction through isocitrate lyase resulting in the release of a succinic acid and a glyoxylic acid. The glyoxylic acid then reacts in a reversible reaction with an acetyl-coa, and a water molecule in a reversible reaction, resulting in a release of a coenzyme A, a hydrogen ion and an L-malic acid. The L-malic acid interacts in a reversible reaction through a NAD driven malate dehydrogenase resulting in the release of NADH, a hydrogen ion and an Oxalacetic acid.
|
Metabolite
Metabolic
|
||
SMP0456855![]() |
Colanic Acid Building Blocks BiosynthesisChlamydia trachomatis D/UW-3/CX
The colonic acid building blocks biosynthesis starts with a Beta-D-Glucose undergoing a transport reaction mediated by a glucose PTS permease. The permease phosphorylates the Beta-D-Glucose, producing a Beta-D-Glucose 6-phosphate. This compound can either change to an Alpha-D-Glucose 6-phosphate spontaneously or into a fructose 6-phosphate through a glucose-6-phosphate isomerase. The latter compound can also be present in E.coli through the interaction of D-fructose and a mannose PTS permease which phosphorylate the D-fructose.
Fructose 6-phosphate interacts in a reversible reaction with mannose-6-phosphate isomerase in order to produce a Alpha-D-mannose 6-phosphate. This compound can also be present in E.coli through the interaction of Alpha-D-mannose and a mannose PTS permease which phosphorylates the alpha-D-mannose. Alpha-D-mannose 6-phosphate interacts in a reversible reaction with a phosphomannomutase to produce a alpha-D-mannose 1-phosphate. This compound in turn with a hydrogen ion and gtp undergoes a reaction with a mannose-1-phosphate guanylyltransferase, releasing a pyrophosphate and producing a guanosine diphosphate mannose. Guanosine diphosphate mannose interacts with gdp-mannose 4,6-dehydratase releasing a water, and gdp-4-dehydro-6-deoxy-D-mannose. This compound in turn with hydrogen ion and NADPH interact with GDP-L-fucose synthase releasing NADP and producing a GDP-L-fucose.
The Alpha-D-Glucose 6-phosphate interacts in a reversible reaction with phosphoglucomutase-1 to produce a alpha-D-glucose 1-phosphate. This in turn with UTP and hydrogen ion interact with UTP--glucose-1-phosphate uridyleltransferase releasing a pyrophosphate and UDP-glucose.
UDP-glucose can either interact with galactose-1-phosphate uridylyltransferase to produce a UDP-galactose or in turn with NAD and water interact with UDP-glucose 6-dehydrogenase releasing a NADH and a hydrogen ion and producing a UDP-glucuronate.
GDP-L-fucose, UDP-glucose, UDP-galactose and UDP-glucuronate are sugars that need to be activated in the form of nucleotide sugar prior to their assembly into colanic acid, also known as M antigen.
Colanic acid is an extracellular polysaccharide which has been linked to a cluster of 19 genes(wca).
|
Metabolite
Metabolic
|
|
|
SMP0456853![]() |
Phospholipid BiosynthesisHaemophilus sputorum CCUG 13788
Phospholipids are membrane components in E. coli. The major phospholipids of E. coli are phosphatidylethanolamine, phosphatidylglycerol, and cardiolipin. All phospholipids contain sn-glycerol-3-phosphate esterified with fatty acids at the sn-1 and sn-2 positions. The reaction starts from a glycerone phosphate (dihydroxyacetone phosphate) produced in glycolysis. The glycerone phosphate is transformed into an sn-glycerol 3-phosphate (glycerol 3 phosphate) by NADPH-driven glycerol-3-phosphate dehydrogenase. sn-Glycerol 3-phosphate is transformed to a 1-acyl-sn-glycerol 3-phosphate (lysophosphatidic acid). This can be achieved by an sn-glycerol-3-phosphate acyltransferase that interacts either with a long-chain acyl-CoA or with an acyl-[acp]. The 1-acyl-sn-glycerol 3-phosphate is transformed into a 1,2-diacyl-sn-glycerol 3-phosphate (phosphatidic acid) through a 1-acylglycerol-3-phosphate O-acyltransferase. This compound is then converted into a CPD-diacylglycerol through a CTP phosphatidate cytididyltransferase. CPD-diacylglycerol can be transformed either into an L-1-phosphatidylserine or an L-1-phosphatidylglycerol-phosphate through a phosphatidylserine synthase or a phosphatidylglycerophosphate synthase, respectively. The L-1-phosphatidylserine transforms into L-1-phosphatidylethanolamine through a phosphatidylserine decarboxylase. On the other hand, L-1-phosphatidylglycerol-phosphate gets transformed into an L-1-phosphatidyl-glycerol through a phosphatidylglycerophosphatase. These 2 products combine to produce a cardiolipin and an ethanolamine. The L-1 phosphatidyl-glycerol can also interact with cardiolipin synthase resulting in a glycerol and a cardiolipin.
|
Metabolite
Metabolic
|
||
SMP0456868![]() |
Purine DegradationHaemophilus sputorum CCUG 13788
Pseudouridine is phosphorylated by interacting with atp and a psuK resulting in the release of an ADP, a hydrogen ion and a pseudouridine 5'-phosphate. The latter compound then reacts with water through a pseudouridine 5'-phosphate glycosidase resulting in the release of a uracil and D-ribofuranose 5-phosphate
|
Metabolite
Metabolic
|
||
SMP0457140![]() |
Phospholipid BiosynthesisPyramidobacter piscolens W5455
Phospholipids are membrane components in E. coli. The major phospholipids of E. coli are phosphatidylethanolamine, phosphatidylglycerol, and cardiolipin. All phospholipids contain sn-glycerol-3-phosphate esterified with fatty acids at the sn-1 and sn-2 positions. The reaction starts from a glycerone phosphate (dihydroxyacetone phosphate) produced in glycolysis. The glycerone phosphate is transformed into an sn-glycerol 3-phosphate (glycerol 3 phosphate) by NADPH-driven glycerol-3-phosphate dehydrogenase. sn-Glycerol 3-phosphate is transformed to a 1-acyl-sn-glycerol 3-phosphate (lysophosphatidic acid). This can be achieved by an sn-glycerol-3-phosphate acyltransferase that interacts either with a long-chain acyl-CoA or with an acyl-[acp]. The 1-acyl-sn-glycerol 3-phosphate is transformed into a 1,2-diacyl-sn-glycerol 3-phosphate (phosphatidic acid) through a 1-acylglycerol-3-phosphate O-acyltransferase. This compound is then converted into a CPD-diacylglycerol through a CTP phosphatidate cytididyltransferase. CPD-diacylglycerol can be transformed either into an L-1-phosphatidylserine or an L-1-phosphatidylglycerol-phosphate through a phosphatidylserine synthase or a phosphatidylglycerophosphate synthase, respectively. The L-1-phosphatidylserine transforms into L-1-phosphatidylethanolamine through a phosphatidylserine decarboxylase. On the other hand, L-1-phosphatidylglycerol-phosphate gets transformed into an L-1-phosphatidyl-glycerol through a phosphatidylglycerophosphatase. These 2 products combine to produce a cardiolipin and an ethanolamine. The L-1 phosphatidyl-glycerol can also interact with cardiolipin synthase resulting in a glycerol and a cardiolipin.
|
Metabolite
Metabolic
|
||
SMP0457152![]() |
Secondary Metabolites: Histidine BiosynthesisAcetomicrobium hydrogeniformans
Histidine biosynthesis starts with a product of PRPP biosynthesis pathway, phosphoribosyl pyrophosphate which interacts with a hydrogen ion through an ATP phosphoribosyltransferase resulting in an pyrophosphate and a phosphoribosyl-ATP. The phosphoribosyl-ATP interacts with water through a phosphoribosyl-AMP cyclohydrolase / phosphoribosyl-ATP pyrophosphatase resulting in the release of pyrophosphate, hydrogen ion and a phosphoribosyl-AMP. The same enzyme proceeds to interact with phosphoribosyl-AMP and water resulting in a 1-(5'-Phosphoribosyl)-5-amino-4-imidazolecarboxamide. The product is then isomerized by a N-(5'-phospho-L-ribosyl-formimino)-5-amino-1-(5'-phosphoribosyl)-4-imidazolecarboxamide isomerase resulting in a PhosphoribosylformiminoAICAR-phosphate, which reacts with L-glutamine through an imidazole glycerol phosphate synthase resulting in a L-glutamic acid, hydrogen ion, 5-aminoimidazole-4-carboxamide and a D-erythro-imidazole-glycerol-phosphate. D-erythro-imidazole-glycerol-phosphate reacts with a imidazoleglycerol-phosphate dehydratase / histidinol-phosphatase, dehydrating the compound and resulting in a imidazole acetol-phosphate. Next, imidazole acetol-phosphate reacts with L-glutamic acid through a histidinol-phosphate aminotransferase, releasing oxoglutaric acid and L-histidinol-phosphate. The latter compound interacts with water and a imidazoleglycerol-phosphate dehydratase / histidinol-phosphatase resulting in L-histidinol and phosphate. L-histidinol interacts with a NAD-driven histidinol dehydrogenase resulting in a Histidinal. Histidinal in turn reacts with water in a NAD driven histidinal dehydrogenase resulting in L-Histidine.
L-Histidine then represses ATP phosphoribosyltransferase, regulation its own biosynthesis.
|
Metabolite
Metabolic
|
|
|
SMP0457147![]() |
Secondary Metabolites: Histidine BiosynthesisBrachyspira pilosicoli B2904
Histidine biosynthesis starts with a product of PRPP biosynthesis pathway, phosphoribosyl pyrophosphate which interacts with a hydrogen ion through an ATP phosphoribosyltransferase resulting in an pyrophosphate and a phosphoribosyl-ATP. The phosphoribosyl-ATP interacts with water through a phosphoribosyl-AMP cyclohydrolase / phosphoribosyl-ATP pyrophosphatase resulting in the release of pyrophosphate, hydrogen ion and a phosphoribosyl-AMP. The same enzyme proceeds to interact with phosphoribosyl-AMP and water resulting in a 1-(5'-Phosphoribosyl)-5-amino-4-imidazolecarboxamide. The product is then isomerized by a N-(5'-phospho-L-ribosyl-formimino)-5-amino-1-(5'-phosphoribosyl)-4-imidazolecarboxamide isomerase resulting in a PhosphoribosylformiminoAICAR-phosphate, which reacts with L-glutamine through an imidazole glycerol phosphate synthase resulting in a L-glutamic acid, hydrogen ion, 5-aminoimidazole-4-carboxamide and a D-erythro-imidazole-glycerol-phosphate. D-erythro-imidazole-glycerol-phosphate reacts with a imidazoleglycerol-phosphate dehydratase / histidinol-phosphatase, dehydrating the compound and resulting in a imidazole acetol-phosphate. Next, imidazole acetol-phosphate reacts with L-glutamic acid through a histidinol-phosphate aminotransferase, releasing oxoglutaric acid and L-histidinol-phosphate. The latter compound interacts with water and a imidazoleglycerol-phosphate dehydratase / histidinol-phosphatase resulting in L-histidinol and phosphate. L-histidinol interacts with a NAD-driven histidinol dehydrogenase resulting in a Histidinal. Histidinal in turn reacts with water in a NAD driven histidinal dehydrogenase resulting in L-Histidine.
L-Histidine then represses ATP phosphoribosyltransferase, regulation its own biosynthesis.
|
Metabolite
Metabolic
|
|
|
SMP0457135![]() |
Secondary Metabolites: Leucine BiosynthesisPaenibacillus lactis 154
Leucine biosynthesis involves a five-step conversion process starting with a 3-methyl-2-oxovaleric acid interacting with acetyl-CoA and a water molecule through a 2-isopropylmalate synthase resulting in Coenzyme A, hydrogen Ion and 2-isopropylmalic acid. The latter compound reacts with isopropylmalate isomerase which dehydrates the compound resulting in a Isopropylmaleate. This compound reacts with water through a isopropylmalate isomerase resulting in 3-isopropylmalate. This compound interacts with a NAD-driven D-malate / 3-isopropylmalate dehydrogenase results in 2-isopropyl-3-oxosuccinate. This compound interacts spontaneously with hydrogen resulting in the release of carbon dioxide and ketoleucine. Ketoleucine interacts in a reversible reaction with L-glutamic acid through a branched-chain amino-acid aminotransferase resulting in Oxoglutaric acid and L-leucine. 2-isopropylmalate synthase and terminal transaminase TyrB can both be suppressed by leucine. 2-keto-isovalerate and tyrosine can both inhibit the TyrB, which lead to absence of IlvE activity. Without IlvE activity, 2-ketoisocaproate could not convert to leucine since branched-chain amino-acid aminotransferase (IlvE) is the only enzyme to facilitate the reaction.
|
Metabolite
Metabolic
|
||
SMP0457156![]() |
Secondary Metabolites: Shikimate PathwayPaenibacillus lactis 154
The biosynthesis of shikimate starts with D-Erythrose-4-phosphate getting transformed into 3-deoxy-D-arabino-heptulosonate-7-phosphate through a phospho-2-dehydro-3-deoxyheptonate aldolase. This is followed by a 3-dehydroquinate synthase converting the 3-deoxy-D-arabino-heptulosonate-7-phosphate into a 3-dehydroquinate which in turn is conveted to 3-dehydroshikimate through a 3-dehydroquinate dehydratase. A this point 3-dehydroshikimate can be turned into Shikimic acid through 2 different reactions involving an NADPH driven Quinate/shikimate dehydrogenase or a NADPH driven shikimate dehydrogenase 2.
Shikimate can also be transported through a shikimate:H+ symporter.
|
Metabolite
Metabolic
|
Showing 375541 -
375550 of 375679 pathways