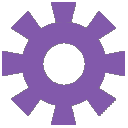
Browsing Pathways
Showing 474711 -
474720 of 605359 pathways
PathBank ID | Pathway Name and Description | Pathway Class | Chemical Compounds | Proteins |
---|---|---|---|---|
SMP0478192![]() |
1,6-Anhydro-N-acetylmuramic Acid RecyclingPrevotella disiens FB035-09AN
Most bacteria, including Escherichia coli, are composed of murein which protects and stabilizes the cell wall. Over half of the murein is broken down by Escherichia coli and recycled for the next generation. The main muropeptide is GlcNAc-anhydro-N-acetylmuramic acid (anhMurNAc)-l-Ala-γ-d-Glu-meso-Dap-d-Ala which enters the cytoplasm by AmpG protein. The peptide is then released from the muropeptide. 1,6-Anhydro-N-acetylmuramic acid (anhMurNAc) is recycled by its conversion to N-acetylglucosamine-phosphate (GlcNAc-P). The sugar is phosphorylated by anhydro-N-acetylmuramic acid kinase (AnmK) to produce MurNAc-P. Etherase cleaves MurNAc-P to produce N-acetyl-D-glucosamine 6-phosphate. The product can undergo further degradation or be recycled into peptidoglycan monomers. The pathway's final product is a peptidoglycan biosynthesis precursor, UDP-N-acetyl-α-D-muramate. The enzyme muropeptide ligase (mpl), attaches the recovered Ala-Glu-DAP tripeptide to the precursor UDP-N-acetyl-α-D-muramate to return to the peptide to the peptidoglycan biosynthetic pathway to synthesize the cell wall.
|
Metabolite
Metabolic
|
|
|
SMP0478208![]() |
Allantoin Degradation (Anaerobic)Prevotella enoeca JCM 12259
Allantoin can be degraded in anaerobic conditions. The first step involves allantoin being degraded by an allantoinase resulting in an allantoate. This compound in turn is metabolized by reacting with water and 2 hydrogen ions through an allantoate amidohydrolase resulting in the release of a carbon dioxide, ammonium and an S-ureidoglycine. The latter compund is further degrades through a S-ureidoglycine aminohydrolase resulting in the release of an ammonium and an S-ureidoglycolate.
S-ureidoglycolate can be metabolized into oxalurate by two different reactions. The first reactions involves a NAD driven ureidoglycolate dehydrogenase resulting in the release of a hydrogen ion , an NADH and a oxalurate. On the other hand S-ureidoglycolate can react with NADP resulting in the release of an NADPH, a hydroge ion and an oxalurate.
It is hypothesized that oxalurate can interact with a phosphate and release a a carbamoyl phosphate and an oxamate.
The carbamoyl phosphate can be further degraded by reacting with an ADP, and a hydrogen ion through a carbamate kinase resulting in the release of an ammonium , ATP and carbon dioxide
|
Metabolite
Metabolic
|
||
SMP0478414![]() |
Salvage Pathways of Pyrimidine DeoxyribonucleotidesAlistipes indistinctus YIT 12060
The pathway begins with the introduction of deoxycytidine into the cytosol, either through a nupG symporter or a nupC symporter. Once inside it is deaminated when reacting with a water molecule, a hydrogen ion and a deoxycytidine deaminase resulting in the release of an ammonium and a deoxyuridine. Deoxyuridine can also be imported through a nupG symporter or a nupC symporter.
Deoxyuridine can react with an ATP through a deoxyuridine kinase resulting in the release of a ADP , a hydrogen ion and a dUMP.
Deoxyuridine can also react with a phosphate through a uracil phosphorylase resulting in the release of a uracil and a deoxy-alpha-D-ribose 1-phosphate. This compound in turn reacts with a thymine through a thymidine phosphorylase resulting in the release of a phosphate and a thymidine. Thymidine in turn reacts with an ATP through a thymidine kinase resulting in a release of an ADP, a hydrogen ion and a dTMP
|
Metabolite
Metabolic
|
||
SMP0478228![]() |
Lipopolysaccharide Biosynthesis IIIPrevotella intermedia ATCC 25611 = DSM 20706
E. coli lipid A is synthesized on the cytoplasmic surface of the inner membrane. The pathway can start from the fructose 6-phosphate that is either produced in the glycolysis and pyruvate dehydrogenase or be obtained from the interaction with D-fructose interacting with a mannose PTS permease. Fructose 6-phosphate interacts with L-glutamine through a D-fructose-6-phosphate aminotransferase resulting into a L-glutamic acid and a glucosamine 6-phosphate. The latter compound is isomerized through a phosphoglucosamine mutase resulting a glucosamine 1-phosphate. This compound is acetylated, interacting with acetyl-CoA through a bifunctional protein glmU resulting in a Coenzyme A, hydrogen ion and N-acetyl-glucosamine 1-phosphate. This compound interact with UTP and hydrogen ion through the bifunctional protein glmU resulting in a pyrophosphate and a UDP-N-acetylglucosamine. This compound interacts with (3R)-3-hydroxymyristoyl-[acp] through an UDP-N-acetylglucosamine acyltransferase resulting in a holo-[acp] and a UDP-3-O[(3R)-3-hydroxymyristoyl]-N-acetyl-alpha-D-glucosamine. This compound interacts with water through UDP-3-O-acyl-N-acetylglucosamine deacetylase resulting in an acetic acid and UDP-3-O-(3-hydroxymyristoyl)-α-D-glucosamine. The latter compound interacts with (3R)-3-hydroxymyristoyl-[acp] through
UDP-3-O-(R-3-hydroxymyristoyl)-glucosamine N-acyltransferase releasing a hydrogen ion, a holo-acp and UDP-2-N,3-O-bis[(3R)-3-hydroxytetradecanoyl]-α-D-glucosamine. The latter compound is hydrolase by interacting with water and a UDP-2,3-diacylglucosamine hydrolase resulting in UMP, hydrogen ion and 2,3-bis[(3R)-3-hydroxymyristoyl]-α-D-glucosaminyl 1-phosphate. This last compound then interacts with a UDP-2-N,3-O-bis[(3R)-3-hydroxytetradecanoyl]-α-D-glucosamine through a lipid A disaccharide synthase resulting in a release of UDP, hydrogen ion and a lipid A disaccharide. The lipid A disaccharide is phosphorylated by an ATP mediated
tetraacyldisaccharide 4'-kinase resulting in the release of hydrogen ion and lipid IVA.
A D-ribulose 5-phosphate is isomerized with D-arabinose 5-phosphate isomerase 2 to result in a D-arabinose 5-phosphate. This compounds interacts with water and phosphoenolpyruvic acid through a 3-deoxy-D-manno-octulosonate 8-phosphate synthase resulting in the release of phosphate and 3-deoxy-D-manno-octulosonate 8-phosphate. This compound interacts with water through a 3-deoxy-D-manno-octulosonate 8-phosphate phosphatase thus releasing a phosphate and a 3-deoxy-D-manno-octulosonate. The latter compound interacts with CTP through a 3-deoxy-D-manno-octulosonate cytidylyltransferase resulting in a pyrophosphate and
CMP-3-deoxy-α-D-manno-octulosonate.
CMP-3-deoxy-α-D-manno-octulosonate and lipid IVA interact with each other through a KDO transferase resulting in CMP, hydrogen ion and alpha-Kdo-(2-->6)-lipid IVA. The latter compound reacts with CMP-3-deoxy-α-D-manno-octulosonate through a KDO transferase resulting in a CMP, hydrogen ion, and a a-Kdo-(2->4)-a-Kdo-(2->6)-lipid IVA. The latter compound can either react with a palmitoleoyl-acp through a palmitoleoyl acyltransferase resulting in the release of a holo-acyl carriere protein and a Kdo2-palmitoleoyl-lipid IVa which in turn reacts with a myristoyl-acp through a myristoyl-acp dependent acyltransferase resulting in a release of a holo-acp and a Kdo2-lipid A, cold adapted, or it can interact with a dodecanoyl-[acp] lauroyl acyltransferase resulting in a holo-[acp] and a (KDO)2-(lauroyl)-lipid IVA. The latter compound reacts with a myristoyl-[acp] through a myristoyl-acyl carrier protein (ACP)-dependent acyltransferase resulting in a holo-[acp], (KDO)2-lipid A. The latter compound reacts with ADP-L-glycero-beta-D-manno-heptose through ADP-heptose:LPS heptosyltransferase I resulting hydrogen ion, ADP, heptosyl-KDO2-lipid A. The latter compound interacts with ADP-L-glycero-beta-D-manno-heptose through ADP-heptose:LPS heptosyltransferase II resulting in ADP, hydrogen ion and (heptosyl)2-Kdo2-lipid A. The latter compound UDP-glucose interacts with (heptosyl)2-Kdo2-lipid A resulting in UDP, hydrogen ion and glucosyl-(heptosyl)2-Kdo2-lipid A. Glucosyl-(heptosyl)2-Kdo2-lipid A (Escherichia coli) is phosphorylated through an ATP-mediated lipopolysaccharide core heptose (I) kinase resulting in ADP, hydrogen ion and glucosyl-(heptosyl)2-Kdo2-lipid A-phosphate.
The latter compound interacts with ADP-L-glycero-beta-D-manno-heptose through a lipopolysaccharide core heptosyl transferase III resulting in ADP, hydrogen ion, and glucosyl-(heptosyl)3-Kdo2-lipid A-phosphate. The latter compound is phosphorylated through an ATP-driven lipopolysaccharide core heptose (II) kinase resulting in ADP, hydrogen ion and glucosyl-(heptosyl)3-Kdo2-lipid A-bisphosphate. The latter compound interacts with UDP-alpha-D-galactose through a UDP-D-galactose:(glucosyl)lipopolysaccharide-1,6-D-galactosyltransferase resulting in a UDP, a hydrogen ion and a galactosyl-glucosyl-(heptosyl)3-Kdo2-lipid A-bisphosphate. The latter compound interacts with UDP-glucose through a (glucosyl)LPS α-1,3-glucosyltransferase resulting in a hydrogen ion, a UDP and galactosyl-(glucosyl)2-(heptosyl)3-Kdo2-lipid A-bisphosphate. This compound then interacts with UDP-glucose through a UDP-glucose:(glucosyl)LPS α-1,2-glucosyltransferase resulting in UDP, a hydrogen ion and galactosyl-(glucosyl)3-(heptosyl)3-Kdo2-lipid A-bisphosphate. This compound then interacts with ADP-L-glycero-beta-D-manno-heptose through a lipopolysaccharide core biosynthesis; heptosyl transferase IV; probably hexose transferase resulting in a Lipid A-core.
A lipid A-core is then exported into the periplasmic space by a lipopolysaccharide ABC transporter.
The lipid A-core is then flipped to the outer surface of the inner membrane by the ATP-binding cassette (ABC) transporter, MsbA. An additional integral membrane protein, YhjD, has recently been implicated in LPS export across the IM. The smallest LPS derivative that supports viability in E. coli is lipid IVA. However, it requires mutations in either MsbA or YhjD, to suppress the normally lethal consequence of an incomplete lipid A . Recent studies with deletion mutants implicate the periplasmic protein LptA, the cytosolic protein LptB, and the IM proteins LptC, LptF, and LptG in the subsequent transport of nascent LPS to the outer membrane (OM), where the LptD/LptE complex flips LPS to the outer surface.
|
Metabolite
Metabolic
|
|
|
SMP0478053![]() |
1,6-Anhydro-N-acetylmuramic Acid RecyclingParaprevotella xylaniphila YIT 11841
Most bacteria, including Escherichia coli, are composed of murein which protects and stabilizes the cell wall. Over half of the murein is broken down by Escherichia coli and recycled for the next generation. The main muropeptide is GlcNAc-anhydro-N-acetylmuramic acid (anhMurNAc)-l-Ala-γ-d-Glu-meso-Dap-d-Ala which enters the cytoplasm by AmpG protein. The peptide is then released from the muropeptide. 1,6-Anhydro-N-acetylmuramic acid (anhMurNAc) is recycled by its conversion to N-acetylglucosamine-phosphate (GlcNAc-P). The sugar is phosphorylated by anhydro-N-acetylmuramic acid kinase (AnmK) to produce MurNAc-P. Etherase cleaves MurNAc-P to produce N-acetyl-D-glucosamine 6-phosphate. The product can undergo further degradation or be recycled into peptidoglycan monomers. The pathway's final product is a peptidoglycan biosynthesis precursor, UDP-N-acetyl-α-D-muramate. The enzyme muropeptide ligase (mpl), attaches the recovered Ala-Glu-DAP tripeptide to the precursor UDP-N-acetyl-α-D-muramate to return to the peptide to the peptidoglycan biosynthetic pathway to synthesize the cell wall.
|
Metabolite
Metabolic
|
|
|
SMP0478133![]() |
Allantoin Degradation (Anaerobic)Prevotella denticola DSM 20614 = JCM 13449
Allantoin can be degraded in anaerobic conditions. The first step involves allantoin being degraded by an allantoinase resulting in an allantoate. This compound in turn is metabolized by reacting with water and 2 hydrogen ions through an allantoate amidohydrolase resulting in the release of a carbon dioxide, ammonium and an S-ureidoglycine. The latter compund is further degrades through a S-ureidoglycine aminohydrolase resulting in the release of an ammonium and an S-ureidoglycolate.
S-ureidoglycolate can be metabolized into oxalurate by two different reactions. The first reactions involves a NAD driven ureidoglycolate dehydrogenase resulting in the release of a hydrogen ion , an NADH and a oxalurate. On the other hand S-ureidoglycolate can react with NADP resulting in the release of an NADPH, a hydroge ion and an oxalurate.
It is hypothesized that oxalurate can interact with a phosphate and release a a carbamoyl phosphate and an oxamate.
The carbamoyl phosphate can be further degraded by reacting with an ADP, and a hydrogen ion through a carbamate kinase resulting in the release of an ammonium , ATP and carbon dioxide
|
Metabolite
Metabolic
|
||
SMP0478436![]() |
Putrescine Degradation IIParabacteroides sp. 20_3
This pathway demonstrates the degradation of extracellular putrescine in E.coli. Putrescine is imported by putrescine transporter (encoded by puuP gene). Putrescine is γ-glutamylated by activation of ATP which generates γ-glutamyl-putrescine, phosphate, and ADP. γ-glutamyl-putrescine is oxidized by gamma-glutamylputrescine oxidoreductase to form γ-glutamyl-γ-butyraldehyde, also produce ammonium and water. Gamma-glutamyl-gamma-aminobutyraldehyde dehydrogenase dehydrogenates γ-glutamyl-γ-butyraldehyde to γ-glutamyl-γ-aminobutyrate, which is then dehydrogenated into γ-Aminobutyric acid and L-Glutamic acid by γ-glutamyl-γ-aminobutyrate hydrolase.
|
Metabolite
Metabolic
|
||
SMP0478443![]() |
Propanoyl-CoA DegradationAlistipes putredinis DSM 17216
The degradation of propanoyl-CoA starts with propanoyl-CoA undergoing a decarboxylase reaction by reacting with hydrogen carbonate and ATP resulting in the release of a phosphate, an ADP, a hydrogen ion and an S-methylmalonyl-CoA. This compound in turn reacts through an epimerase reaction resulting in the release of a R-methylmalonyl-CoA. This compound in turn can undergo a reversible reaction through a methylmalonyl-CoA mutase resulting in the release of a succinyl-CoA. This compound can be converted back to R-methylmalonyl-CoA through a methylmalonyl-CoA mutase.
Methylmalonyl-CoA can then be converted into propanoyl-CoA through a methylmalonyl CoA decarboxylase . This compound in turn reacts with a succinate through a propionyl-CoA succinate CoA transferase resulting in the release of a propanoate and a succinyl-CoA.
|
Metabolite
Metabolic
|
||
SMP0478454![]() |
Salvage Pathways of Pyrimidine DeoxyribonucleotidesParabacteroides sp. 20_3
The pathway begins with the introduction of deoxycytidine into the cytosol, either through a nupG symporter or a nupC symporter. Once inside it is deaminated when reacting with a water molecule, a hydrogen ion and a deoxycytidine deaminase resulting in the release of an ammonium and a deoxyuridine. Deoxyuridine can also be imported through a nupG symporter or a nupC symporter.
Deoxyuridine can react with an ATP through a deoxyuridine kinase resulting in the release of a ADP , a hydrogen ion and a dUMP.
Deoxyuridine can also react with a phosphate through a uracil phosphorylase resulting in the release of a uracil and a deoxy-alpha-D-ribose 1-phosphate. This compound in turn reacts with a thymine through a thymidine phosphorylase resulting in the release of a phosphate and a thymidine. Thymidine in turn reacts with an ATP through a thymidine kinase resulting in a release of an ADP, a hydrogen ion and a dTMP
|
Metabolite
Metabolic
|
||
SMP0478459![]() |
Thiosulfate Disproportionation IIIParabacteroides johnsonii DSM 18315
Thiosulfate sulfurtransferase (also known as rhodanese) can facilitate the transfer of a sulfur atom from sulfur donors to nucleophilic sulfur acceptors, and it has been found in many major phyla (prokaryotic and eukaryotic). The role of thiosulfate sulfurtransferase might be the detoxification of cyanide in both bacteria and mammals, or it might also involve in formation of prosthetic groups in iron-sulfur proteins. In this pathway, thiosulfate and hydrogen cyanide have been catalyzed by thiosulfate sulfurtransferase to form thiocyanate and sulfite. Sulfite is used in later sulfur metabolism.
|
Metabolite
Metabolic
|
Showing 474711 -
474720 of 476962 pathways