Page not found.
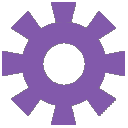
Loading Pathway...
Error: Pathway image not found.
Hide
Pathway Description
Tryptophan Metabolism
Bos taurus
Category:
Metabolite Pathway
Sub-Category:
Metabolic
Created: 2018-08-10
Last Updated: 2023-10-29
This pathway depicts the metabolic reactions and pathways associated with tryptophan metabolism in animals. Tryptophan is an essential amino acid. This means that it cannot be synthesized by humans and other mammals and therefore must be part of the diet. Unlike animals, plants and microbes can synthesize tryptophan from shikimic acid or anthranilate. As one of the 20 proteogenic amino acids, tryptophan plays an important role in protein biosynthesis through the action of tryptophanyl-tRNA synthetase. As shown in this pathway, tryptophan can be linked to the tryptophanyl-tRNA via either the mitochondrial or cytoplasmic tryptophan tRNA ligases. Also shown in this pathway map is the conversion of tryptophan to serotonin (a neurotransmitter). In this process, tryptophan is acted upon by the enzyme tryptophan hydroxylase, which produces 5-hydroxytryptophan (5HTP). 5HTP is then converted into serotonin (5-HT) via aromatic amino acid decarboxylase. Serotonin, in turn, can be converted into N-acetyl serotonin (via serotonin-N-acetyltransferase) and then melatonin (a neurohormone), via 5-hydroxyindole-O-methyltransferase. The melatonin can be converted into 6-hydroxymelatonin via the action of cytochrome P450s in the endoplasmic reticulum. Serotonin has other fates as well. As depicted in this pathway it can be converted into N-methylserotonin via Indolethylamine-N-methyltransferase (INMT) or it can be converted into formyl-5-hydroxykynurenamine via indoleamine 2,3-dioxygenase. Serotonin may also be converted into 5-methoxyindoleacetate via a series of intermediates including 5-hydroxyindoleacetaldehyde and 5-hydroxyindoleacetic acid. Tryptophan can be converted or broken down into many other compounds as well. It can be converted into tryptamine via the action of aromatic amino acid decarboxylase. The resulting tryptamine can then be converted into indoleacetaldehyde via kynurenine 3-monooxygenase and then into indoleacetic acid via the action of aldehyde dehydrogenase. Tryptophan also leads to the production of a very important compound known as kynurenine. Kynurenine is synthesized via the action of tryptophan 2,3-dioxygnase, which produces N-formylkynurenine. This compound is converted into kynurenine via the enzyme known as kynurenine formamidase (AFMID). Kynurenine has at least 3 fates. First, kynurenine can undergo deamination in a standard transamination reaction yielding kynurenic acid. Secondly, kynurenine can undergo a series of catabolic reactions (involving kynureninase and kynurenine 3-monooxygenase) producing 3-hydroxyanthranilate plus alanine. In this reaction, kynureninase catabolizes the conversion of kynurenine into anthranilic acid while kynurenine—oxoglutarate transaminase (also known as kynurenine aminotransferase or glutamine transaminase K, GTK) catabolizes its conversion into kynurenic acid. The action of kynurenine 3-hydroxylase on kynurenic acid leads to 3-hydroxykynurenine. The oxidation of 3-hydroxyanthranilate converts it into 2-amino-3-carboxymuconic 6-semialdehyde, which has two fates. It can either degrade to form acetoacetate or it can cyclize to form quinolate. Most of the body’s 3-hydroxyanthranilate leads to the production of acetoacetate (a ketone body), which is why tryptophan is also known as a ketogenic amino acid. An important side reaction in the liver involves a non-enzymatic cyclization into quinolate followed by transamination and several rearrangements to yield limited amounts of nicotinic acid, which leads to the production of a small amount of NAD+ and NADP+.
References
Tryptophan Metabolism References
Zimin AV, Delcher AL, Florea L, Kelley DR, Schatz MC, Puiu D, Hanrahan F, Pertea G, Van Tassell CP, Sonstegard TS, Marcais G, Roberts M, Subramanian P, Yorke JA, Salzberg SL: A whole-genome assembly of the domestic cow, Bos taurus. Genome Biol. 2009;10(4):R42. doi: 10.1186/gb-2009-10-4-r42. Epub 2009 Apr 24.
Pubmed: 19393038
Harhay GP, Sonstegard TS, Keele JW, Heaton MP, Clawson ML, Snelling WM, Wiedmann RT, Van Tassell CP, Smith TP: Characterization of 954 bovine full-CDS cDNA sequences. BMC Genomics. 2005 Nov 23;6:166. doi: 10.1186/1471-2164-6-166.
Pubmed: 16305752
Schroeder WA, Shelton JR, Shelton JB, Robberson B, Apell G, Fang RS, Bonaventura J: The complete amino acid sequence of bovine liver catalase and the partial sequence of bovine erythrocyte catalase. Arch Biochem Biophys. 1982 Mar;214(1):397-421. doi: 10.1016/0003-9861(82)90044-3.
Pubmed: 7082009
Murthy MR, Reid TJ 3rd, Sicignano A, Tanaka N, Rossmann MG: Structure of beef liver catalase. J Mol Biol. 1981 Oct 25;152(2):465-99. doi: 10.1016/0022-2836(81)90254-0.
Pubmed: 7328661
Ko TP, Day J, Malkin AJ, McPherson A: Structure of orthorhombic crystals of beef liver catalase. Acta Crystallogr D Biol Crystallogr. 1999 Aug;55(Pt 8):1383-94. doi: 10.1107/s0907444999007052.
Pubmed: 10417406
Dilovic I, Gliubich F, Malpeli G, Zanotti G, Matkovic-Calogovic D: Crystal structure of bovine 3-hydroxyanthranilate 3,4-dioxygenase. Biopolymers. 2009 Dec;91(12):1189-95. doi: 10.1002/bip.21167.
Pubmed: 19226621
Kang UJ, Joh TH: Deduced amino acid sequence of bovine aromatic L-amino acid decarboxylase: homology to other decarboxylases. Brain Res Mol Brain Res. 1990 Jun;8(1):83-7. doi: 10.1016/0169-328x(90)90013-4.
Pubmed: 2166204
Guan KL, Weiner H: Sequence of the precursor of bovine liver mitochondrial aldehyde dehydrogenase as determined from its cDNA, its gene, and its functionality. Arch Biochem Biophys. 1990 Mar;277(2):351-60. doi: 10.1016/0003-9861(90)90590-u.
Pubmed: 1689984
Farres J, Guan KL, Weiner H: Primary structures of rat and bovine liver mitochondrial aldehyde dehydrogenases deduced from cDNA sequences. Eur J Biochem. 1989 Mar 1;180(1):67-74. doi: 10.1111/j.1432-1033.1989.tb14616.x.
Pubmed: 2540003
Lee JE, Cho YD: Purification and characterization of bovine brain gamma-aminobutyraldehyde dehydrogenase. Biochem Biophys Res Commun. 1992 Nov 30;189(1):450-4.
Pubmed: 1449496
Ishida I, Obinata M, Deguchi T: Molecular cloning and nucleotide sequence of cDNA encoding hydroxyindole O-methyltransferase of bovine pineal glands. J Biol Chem. 1987 Feb 25;262(6):2895-9.
Pubmed: 3818627
Donohue SJ, Roseboom PH, Klein DC: Bovine hydroxyindole-O-methyltransferase. Significant sequence revision. J Biol Chem. 1992 Mar 15;267(8):5184-5.
Pubmed: 1544900
Bernard M, Donohue SJ, Klein DC: Human hydroxyindole-O-methyltransferase in pineal gland, retina and Y79 retinoblastoma cells. Brain Res. 1995 Oct 23;696(1-2):37-48. doi: 10.1016/0006-8993(95)00651-6.
Pubmed: 8574683
This pathway was propagated using PathWhiz -
Pon, A. et al. Pathways with PathWhiz (2015) Nucleic Acids Res. 43(Web Server issue): W552–W559.
Propagated from SMP0000063
Highlighted elements will appear in red.
Highlight Compounds
Highlight Proteins
Enter relative concentration values (without units). Elements will be highlighted in a color gradient where red = lowest concentration and green = highest concentration. For the best results, view the pathway in Black and White.
Visualize Compound Data
Visualize Protein Data
Downloads
Settings