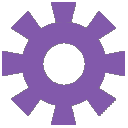
Browsing Pathways
Showing 21 -
30 of 605359 pathways
PathBank ID | Pathway Name and Description | Pathway Class | Chemical Compounds | Proteins |
---|---|---|---|---|
SMP0000023 |
Steroid BiosynthesisHomo sapiens
The steroid biosynthesis (or cholesterol biosynthesis) pathway is an anabolic metabolic pathway that produces steroids from simple precursors. It starts with the mevalonate pathway, where acetyl-CoA and acetoacetyl-CoA are the first two building blocks. These compounds are joined together via the enzyme hydroxy-3-methylgutaryl (HMG)-CoA synthase to produce the compound known as hydroxy-3-methylgutaryl-CoA (HMG-CoA). This compound is then reduced to mevalonic acid via the enzyme HMG-CoA reductase. It is important to note that HMG-CoA reductase is the protein target of many cholesterol-lowering drugs called statins (PMID: 12602122). The resulting mevalonic acid (or mevalonate) is then phosphorylated by the enzyme known as mevalonate kinase to form mevalonate-5-phosphate, which is then phosphorylated again by phosphomevalonate kinase to form mevolonate-5-pyrophsophate. This pyrophosphorylated compound is subsequently decarboxylated via the enzyme mevolonate-5-pyrophsophate decarboxylase to form isopentylpyrophosphate (IPP). IPP can also be isomerized (via isopentenyl-PP-isomerase) to form dimethylallylpyrophosphate (DMAPP). IPP and DMAPP can both donate isoprene units, which can then be joined together to make farnesyl and geranylgeranyl intermediates. Specifically, three molecules of IPP condense to form farnesyl pyrophosphate through the action of the enzyme known as geranyl transferase. Two molecules of farnesyl pyrophosphate then condense to form a molecule known as squalene by the action of the enzyme known as squalene synthase in the cell’s endoplasmic reticulum. The enzyme oxidosqualene cyclase then cyclizes squalene to form lanosterol. Lanosterol is a tetracyclic triterpenoid, and serves as the framework from which all steroids are derived. 14-Demethylation of lanosterol by a cytochrome P450 enzyme known as CYP51 eventually yields cholesterol. Cholesterol is the central steroid in human biology. It can be obtained from animal fats consumed in the diet or synthesized de novo (as described above). Cholesterol is an essential constituent of lipid bilayer membranes (where it forms cholesterol esters) and is the starting point for the biosynthesis of steroid hormones, bile acids and bile salts, and vitamin D. Steroid hormones are mostly synthesized in the adrenal gland and gonads. They regulate energy metabolism and stress responses (via glucocorticoids such as cortisol), salt balance (mineralocorticoids such as aldosterone), and sexual development and function (via androgens such as testosterone and estrogens such as estradiol). Bile acids and bile salts (such as taurocholate) are mostly synthesized in the liver. They are released into the intestine and function as detergents to solubilize dietary fats. Cholesterol is the main constituent of atheromas. These are the fatty lumps found in the walls of arteries that occur in atherosclerosis and, when ruptured, can cause heart attacks.
|
Metabolite
Metabolic
|
|
|
SMP0000024 |
Porphyrin MetabolismHomo sapiens
This pathway depicts the synthesis and breakdown of porphyrin. The porphyrin ring is the framework for the heme molecule, the pigment in hemoglobin and red blood cells. The first reaction in porphyrin ring biosynthesis takes place in the mitochondria and involves the condensation of glycine and succinyl-CoA by delta-aminolevulinic acid synthase (ALAS). Delta-aminolevulinic acid (ALA) is also called 5-aminolevulinic acid. Following its synthesis, ALA is transported into the cytosol, where ALA dehydratase (also called porphobilinogen synthase) dimerizes 2 molecules of ALA to produce porphobilinogen. The next step in the pathway involves the condensation of 4 molecules of porphobilinogen to produce hydroxymethylbilane (also known as HMB). The enzyme that catalyzes this condensation is known as porphobilinogen deaminase (PBG deaminase). This enzyme is also called hydroxymethylbilane synthase or uroporphyrinogen I synthase. Hydroxymethylbilane (HMB) has two main fates. Most frequently it is enzymatically converted into uroporphyrinogen III, the next intermediate on the path to heme. This step is mediated by two enzymes: uroporphyrinogen synthase and uroporphyrinogen III cosynthase. Hydroxymethylbilane can also be non-enzymatically cyclized to form uroporphyrinogen I. In the cytosol, the uroporphyrinogens (uroporphyrinogen III or uroporphyrinogen I) are decarboxylated by the enzyme uroporphyrinogen decarboxylase. These new products have methyl groups in place of the original acetate groups and are known as coproporphyrinogens. Coproporphyrinogen III is the most important intermediate in heme synthesis. Coproporphyrinogen III is transported back from the cytosol into the interior of the mitochondria, where two propionate residues are decarboxylated (via coproporphyrinogen-III oxidase), which results in vinyl substituents on the 2 pyrrole rings. The resulting product is called protoporphyrinogen IX. The protoporphyrinogen IX is then converted into protoporphyrin IX by another enzyme called protoporphyrinogen IX oxidase. The final reaction in heme synthesis also takes place within the mitochondria and involves the insertion of the iron atom into the ring system generating the molecule known heme b. The enzyme catalyzing this reaction is known as ferrochelatase. The largest repository of heme in the body is in red blood cells (RBCs). RBCs have a life span of about 120 days. When the RBCs have reached the end of their useful lifespan, the cells are engulfed by macrophages and their constituents recycled or disposed of. Heme is broken down when the heme ring is opened by the enzyme known as heme oxygenase, which is found in the endoplasmic reticulum of the macrophages. The oxidation process produces the linear tetrapyrrole biliverdin, ferric iron (Fe3+), and carbon monoxide (CO). The carbon monoxide (which is toxic) is eventually discharged through the lungs. In the next reaction, a second methylene group (located between rings III and IV of the porphyrin ring) is reduced by the enzyme known as biliverdin reductase, producing bilirubin. Bilirubin is significantly less extensively conjugated than biliverdin. This reduction causes a change in the colour of the molecule from blue-green (biliverdin) to yellow-red (bilirubin). In hepatocytes, bilirubin-UDP-glucuronyltransferase (bilirubin-UGT) adds two additional glucuronic acid molecules to bilirubin to produce the more water-soluble version of the molecule known as bilirubin diglucuronide. In most individuals, intestinal bilirubin is acted on by the gut bacteria to produce the final porphyrin products, urobilinogens and stercobilins. These are excreted in the feces. The stercobilins oxidize to form brownish pigments which lead to the characteristic brown colour found in normal feces. Some of the urobilinogen produced by the gut bacteria is reabsorbed and re-enters the circulation. These urobilinogens are converted into urobilins that are then excreted in the urine which cause the yellowish colour in urine.
|
Metabolite
Metabolic
|
|
|
SMP0000025 |
Phospholipid BiosynthesisHomo sapiens
This pathway describes the synthesis of the common phospholipids, including phosphatidylcholine, phosphatidylethanolamine, phosphatidylserine, phosphatidylinositol and cardiolipins. Phospholipid synthesis is mediated by two possible mechanisms: (1) A CDP-activated polar head group for attaches to the phosphate of phosphatidic acid or (2) A CDP-activated 1,2-diacylglycerol and an inactivated polar head group. The ER membrane is the primary site of phospholipid synthesis using precursors imported into the ER from the cytosol. To initiate the process, phosphatidic acid is generated by the linkage of two fatty acids associated with coenzyme A (CoA) carriers to glycerol-3-phosphate. This new molecule is inserted into the membrane where a phosphatase converts it into diacylglycerol or alternatively it is formed into phosphatidylinositol before the conversion. If the conversion into diacylglycerol occurs, the molecule has three possible fates depending on the type of polar head group attached: phosphatidylcholine, phosphatidylethanolamine, or phosphatidylserine.
At their inception, a phospholipid is composed of a saturated fatty acid and unsaturated fatty acid on the C1 and C2 carbon of the glycerol backbone respectively. With the continuous remodelling of the phospholipid bilayer, this fatty acid distribution at these carbons changes. For example, acyl group remodelling changes the presence of acyl groups on the glycerol backbone (which were initially placed there by acyl transferases) and moves it further into the membrane as a consequence of the action of phospholipase A1 (PLA1) and phospholipase A2 (PLA2). Another modifying group that is usually added are alcohol-containing groups such as serine, ethanol amine, and choline which contain positively-charged nitrogen.
|
Metabolite
Metabolic
|
|
|
SMP0000027 |
Pantothenate and CoA BiosynthesisHomo sapiens
Pantothenate, also called vitamin B5, is a nutrient that everyone requires in their diet. The nutrient gets its name from the greek word “pantothen” which means “from everywhere.” The reason it is called this is because pantothenic acid is found in almost every food. It is a precursor of coenzyme A, which is an essential part of many reactions in the body, specifically important in the production of compounds like cholesterol and different fatty acids. Most of pantothenic acid is found in food as phosphopentetheine or coenzyme A. Pantothenic acid, pantetheine 4’-phosphate and pantetheine are all found in red blood cells. The 6 step process in which coenzyme A is created begins with the creation of pantothenic acid from pantetheine, which is catalyzed by the enzyme pantetheinase. Pantothenic acid then works with pantothenate kinase 1 to produce D-4’-phosphopantothenate. This compound quickly becomes 4’phosphopantothenoylcysteine through the enzyme phosphopantothenate-cysteine ligase. 4’phosphopantothenoylcysteine then uses phosphopantothenoylcysteine decarboxylase to create pantetheine 4’-phosphate. This compound then undergoes two reactions, both resulting in the production of dephospho-CoA; the first reaction uses ectonucleotide pyrophosphatase/phosphodiesterase family member 1, the second uses bifunctional coenzyme A synthase. In the final step of coenzyme A synthesization, bifunctional coenzyme A synthase catalyzes dephospho-CoA into coenzyme A.
|
Metabolite
Metabolic
|
|
|
SMP0000028 |
Caffeine MetabolismHomo sapiens
Caffeine is obtained from diet including coffee and other beverages and is absorbed in the stomach and small intestine. In the liver, the cytochrome P450 oxidase enzyme system and specifically CYP1A2 metabolizes caffeine into paraxanthine to increase lipolysis and increase free fatty acids and glycerol levels in the blood, theobromine to dilate blood vessels and increase urine volume and theophylline which relaxes bronchi smooth muscles. In the lysosome, these metabolites undergo further metabolism into methyluric acids before being excreted in the urine. There is genetic variability in the metabolism of caffeine due to the polymorphism of CYP1A2. This variability can affect the pharmacokinetic and pharmacodynamic properties of caffeine and may affect an individual's consumption.
|
Metabolite
Metabolic
|
|
|
SMP0000029 |
Selenoamino Acid MetabolismHomo sapiens
Phospholipids are membrane components in P. aeruginosa. The major phospholipids of P. aeruginosa are phosphatidylethanolamine, phosphatidylglycerol, and cardiolipin. All phospholipids contain sn-glycerol-3-phosphate esterified with fatty acids at the sn-1 and sn-2 positions. The reaction starts from a glycerone phosphate (dihydroxyacetone phosphate) produced in glycolysis. The glycerone phosphate is transformed into an sn-glycerol 3-phosphate (glycerol 3 phosphate) by NADPH-driven glycerol-3-phosphate dehydrogenase. sn-Glycerol 3-phosphate is transformed to a 1-acyl-sn-glycerol 3-phosphate (lysophosphatidic acid). This can be achieved by an sn-glycerol-3-phosphate acyltransferase that interacts either with a long-chain acyl-CoA or with an acyl-[acp]. The 1-acyl-sn-glycerol 3-phosphate is transformed into a 1,2-diacyl-sn-glycerol 3-phosphate (phosphatidic acid) through a 1-acylglycerol-3-phosphate O-acyltransferase. This compound is then converted into a CPD-diacylglycerol through a CTP phosphatidate cytididyltransferase. CPD-diacylglycerol can be transformed either into an L-1-phosphatidylserine or an L-1-phosphatidylglycerol-phosphate through a phosphatidylserine synthase or a phosphatidylglycerophosphate synthase, respectively. The L-1-phosphatidylserine transforms into L-1-phosphatidylethanolamine through a phosphatidylserine decarboxylase. On the other hand, L-1-phosphatidylglycerol-phosphate gets transformed into an L-1-phosphatidyl-glycerol through a phosphatidylglycerophosphatase. These 2 products combine to produce a cardiolipin and an ethanolamine. The L-1 phosphatidyl-glycerol can also interact with cardiolipin synthase resulting in a glycerol and a cardiolipin.
|
Metabolite
Metabolic
|
|
|
SMP0000030 |
Oxidation of Branched-Chain Fatty AcidsHomo sapiens
In the majority of organisms, fatty acid degradation occurs mostly through the beta-oxidation cycle. In plants, this cycle only happens in the peroxisome, while in mammals this cycle happens in both the peroxisomes and mitochondria. Unfortunately, traditional fatty acid oxidation does not work for branched-chain fatty acids, or fatty acids that do not have an even number of carbons, like the fatty acid phytanic acid, found in animal milk. This acid can not be oxidized through beta-oxidation, as problems arise when water is added at the branched beta-carbon. To be able to oxidize this fatty acid, the carbon is oxidized by oxygen, which removes the initial carboxyl group, which shortens the chain. Now lacking a methyl group, this chain can be beta-oxidized. Now moving to the mitochondria, there are four reactions that occur, and are repeated for each molecule of the fatty acid. Each time the cycle of these reactions is completed, the chain is relieved of two carbons, which are oxidized and are taken away by NADH and FADH2, energy carriers that collect the carbons energy. After beta-oxidation in the cycle of reactions, an acetyl-CoA unit is released and is recycled into the cycle of reactions in the mitochondria, until the chain is fully broken down into acetyl-CoA, and can enter the TCA cycle. Once in the TCA cycle, it is converted to NADH and FADH2, which in turn help move along mitochondrial ATP production. Acetyl-CoA also helps produce ketone bodies that are further converted to energy in the heart and the brain.
|
Metabolite
Metabolic
|
|
|
SMP0000031 |
Pentose Phosphate PathwayHomo sapiens
The pentose phosphate pathway—also referred to in the literature as the phosphogluconate pathway, the hexose monophosphate shunt, or the pentose phosphate shunt—is involved in the generation of NADPH as well as pentose sugars. Of the total cytoplasmic NADPH used in biosynthetic reactions, a significant proportion of it is generated through the pentose phosphate pathway. Ribose 5-phosphate is also another essential product generated by this pathway which is employed in nucleotide synthesis. The pentose phosphate pathway is also involved in the digestive process as the products of nucleic acid catabolism can be metabolized through the pathway (pentose sugars are usually yielded in the breakdown) while the carbon backbones of dietary carbohydrates can be converted into glycolytic/gluconeogenic intermediates. The pentose phosphate pathway is interconnected to the glycolysis pathway through the shared use of three intermediates: glucose 6-phosphate, glyceraldehyde 3-phosphate, and fructose 6-phosphate.
The pathway can be described as eight distinct reactions (see below) and is separated into an oxidative phase and a non-oxidative phase. Reactions 1-3 form the oxidative phase and generate NADPH and pentose 5-phosphate. Reactions 4-8 form the non-oxidative phase and converts pentose 5-phosphate into other pentose sugars such as ribose 5-phosphate, but generates no NADPH. The eight reactions are as follows: reaction 1 where glucose-6-phosphate 1-dehydrogenase converts glucose 6-phosphate into D-glucono-1,5-lactone 6-phosphate with NADPH formation; reaction 2 where 6-phosphogluconolactonase converts D-glucono-1,5-lactone 6-phosphate into 6-phospho-D-gluconate;reaction 3 where 6-phosophogluconate dehydrogenase converts 6-phospho-D-gluconate into ribulose 5-phosphate with NADPH formation; reaction 4 where ribulose-phosphate 3-epimerase converts ribulose 5-phosphate into xylulose 5-phosphate; reaction 5 where ribose-5-phosphate isomerase converts ribulose 5-phosphate into ribose 5-phosphate; reaction 6 where transketolase rearranges ribose 5-phosphate and xylulose 5-phosphate to form sedoheptulose 7-phosphate and glyceraldehyde 3-phosphate; reaction 7 where transaldolase rearranges of sedoheptulose 7-phosphate and glyceraldehyde 3-phosphate to form erythrose 4-phosphate and fructose 6-phosphate; and reaction 8 where transkelotase rearranges of xylulose 5-phosphate and erythrose 4-phosphate to form glyceraldehyde 3-phosphate and fructose-6-phosphate.
|
Metabolite
Metabolic
|
|
|
SMP0000032 |
Valine, Leucine, and Isoleucine DegradationHomo sapiens
Valine, isoleuciine, and leucine are essential amino acids and are identified as the branched-chain amino acids (BCAAs). The catabolism of all three amino acids starts in muscle and yields NADH and FADH2 which can be utilized for ATP generation. The catabolism of all three of these amino acids uses the same enzymes in the first two steps. The first step in each case is a transamination using a single BCAA aminotransferase, with α-ketoglutarate as the amine acceptor. As a result, three different α-keto acids are produced and are oxidized using a common branched-chain α-keto acid dehydrogenase (BCKD), yielding the three different CoA derivatives. Isovaleryl-CoA is produced from leucine by these two reactions, alpha-methylbutyryl-CoA from isoleucine, and isobutyryl-CoA from valine. These acyl-CoA’s undergo dehydrogenation, catalyzed by three different but related enzymes, and the breakdown pathways then diverge. Leucine is ultimately converted into acetyl-CoA and acetoacetate; isoleucine into acetyl-CoA and succinyl-CoA; and valine into propionyl-CoA (and subsequently succinyl-CoA). Under fasting conditions, substantial amounts of all three amino acids are generated by protein breakdown. In muscle, the final products of leucine, isoleucine, and valine catabolism can be fully oxidized via the citric acid cycle; in the liver, they can be directed toward the synthesis of ketone bodies (acetoacetate and acetyl-CoA) and glucose (succinyl-CoA). Because isoleucine catabolism terminates with the production of acetyl-CoA and propionyl-CoA, it is both glucogenic and ketogenic. Because leucine gives rise to acetyl-CoA and acetoacetyl-CoA, it is classified as strictly ketogenic.
|
Metabolite
Metabolic
|
|
|
SMP0000033 |
Methionine MetabolismHomo sapiens
Methionine metabolism is a process that is necessary for humans. Methionine metabolism in mammals happens within two pathways, a methionine cycle and a transsulfuration sequence. These pathways have three common reactions with both pathways including the transformation of methionine to S-adenosylmethionine (SAM), the use of SAM in many different transmethylation reactions resulting in a methylated product plus S-adenosylhomocysteine, and the conversion of S-adenosylhomocysteine to produce the compounds homocysteine and adenosine. The reactions mentioned above not only produce cysteine, they also create a-ketobutyrate. This compound is then converted to succinyl-CoA through a three step process after being converted to propionyl-CoA. If the amino acids cysteine and methionine are available in enough quantity, the pathway will accumulate SAM and this will in turn encourage the production of cysteine and a-ketobutyrate, which are both glucogenic, through cystathionine synthase. When there is a lack of methionine, there is a decrease in the production of SAM, which limits cystathionine synthase activity.
|
Metabolite
Metabolic
|
|
|
Showing 21 -
30 of 526090 pathways