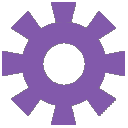
Loading Pathway...
Error: Pathway image not found.
Hide
Pathway Description
Galactose Degradation/Leloir Pathway
Escherichia coli
Category:
Metabolite Pathway
Sub-Category:
Metabolic
Created: 2015-05-01
Last Updated: 2025-05-31
The degradation of galactose, also known as Leloir pathway, requires 3 main enzymes once Beta-D-galactose has been converted to galactose through an Aldose-1-epimerase. These are: galactokinase , galactose-1-phosphate uridylyltransferase and UDP-glucose 4-epimerase. Beta-D-galactose can be uptaken from the environment through a galactose proton symporter. It can also be produced by lactose degradation involving a lactose permease to uptake lactose from the environment and a beta-galactosidase to turn lactose into Beta-D-galactose.
Galactose is degraded through the following process:
Beta-D-galactose is introduced into the cytoplasm through a galactose proton symporter, or it can be synthesized from an alpha lactose that is introduced into the cytoplasm through a lactose permease. Alpha lactose interacts with water through a beta-galactosidase resulting in a beta-D-glucose and beta-D-galactose. Beta-D-galactose is isomerized into D-galactose. D-Galactose undergoes phosphorylation through a galactokinase, hence producing galactose 1 phosphate. On the other side of the pathway, a gluose-1-phosphate (product of the interaction of alpha-D-glucose 6-phosphate with a phosphoglucomutase resulting in a alpha-D-glucose-1-phosphate, an isomer of Glucose 1-phosphate, or an isomer of Beta-D-glucose 1-phosphate) interacts with UTP and a hydrogen ion in order to produce a uridine diphosphate glucose. This is followed by the interaction of galactose-1-phosphate with an established amount of uridine diphosphate glucose through a galactose-1-phosphate uridylyltransferase, which in turn output a glucose-1-phosphate and a uridine diphosphate galactose. The glucose -1-phosphate is transformed into a uridine diphosphate glucose through UTP--glucose-1-phosphate uridylyltransferase. The product, uridine diphosphate glucose, can undergo a reversible reaction in which it can be turned into uridine diphosphategalactose through an UDP-glucose 4-epimerase, and so the cycle can keep going as long as more lactose or galactose is imported into the cell.
References
Galactose Degradation/Leloir Pathway References
Frey PA: The Leloir pathway: a mechanistic imperative for three enzymes to change the stereochemical configuration of a single carbon in galactose. FASEB J. 1996 Mar;10(4):461-70.
Pubmed: 8647345
Ueguchi C, Ito K: Multicopy suppression: an approach to understanding intracellular functioning of the protein export system. J Bacteriol. 1992 Mar;174(5):1454-61. doi: 10.1128/jb.174.5.1454-1461.1992.
Pubmed: 1537791
Weissborn AC, Liu Q, Rumley MK, Kennedy EP: UTP: alpha-D-glucose-1-phosphate uridylyltransferase of Escherichia coli: isolation and DNA sequence of the galU gene and purification of the enzyme. J Bacteriol. 1994 May;176(9):2611-8. doi: 10.1128/jb.176.9.2611-2618.1994.
Pubmed: 8169209
Hossain SA, Tanizawa K, Kazuta Y, Fukui T: Overproduction and characterization of recombinant UDP-glucose pyrophosphorylase from Escherichia coli K-12. J Biochem. 1994 May;115(5):965-72. doi: 10.1093/oxfordjournals.jbchem.a124446.
Pubmed: 7961613
Debouck C, Riccio A, Schumperli D, McKenney K, Jeffers J, Hughes C, Rosenberg M, Heusterspreute M, Brunel F, Davison J: Structure of the galactokinase gene of Escherichia coli, the last (?) gene of the gal operon. Nucleic Acids Res. 1985 Mar 25;13(6):1841-53. doi: 10.1093/nar/13.6.1841.
Pubmed: 3158881
Oshima T, Aiba H, Baba T, Fujita K, Hayashi K, Honjo A, Ikemoto K, Inada T, Itoh T, Kajihara M, Kanai K, Kashimoto K, Kimura S, Kitagawa M, Makino K, Masuda S, Miki T, Mizobuchi K, Mori H, Motomura K, Nakamura Y, Nashimoto H, Nishio Y, Saito N, Horiuchi T, et al.: A 718-kb DNA sequence of the Escherichia coli K-12 genome corresponding to the 12.7-28.0 min region on the linkage map. DNA Res. 1996 Jun 30;3(3):137-55. doi: 10.1093/dnares/3.3.137.
Pubmed: 8905232
Blattner FR, Plunkett G 3rd, Bloch CA, Perna NT, Burland V, Riley M, Collado-Vides J, Glasner JD, Rode CK, Mayhew GF, Gregor J, Davis NW, Kirkpatrick HA, Goeden MA, Rose DJ, Mau B, Shao Y: The complete genome sequence of Escherichia coli K-12. Science. 1997 Sep 5;277(5331):1453-62. doi: 10.1126/science.277.5331.1453.
Pubmed: 9278503
Bouffard GG, Rudd KE, Adhya SL: Dependence of lactose metabolism upon mutarotase encoded in the gal operon in Escherichia coli. J Mol Biol. 1994 Dec 2;244(3):269-78. doi: 10.1006/jmbi.1994.1728.
Pubmed: 7966338
Kalnins A, Otto K, Ruther U, Muller-Hill B: Sequence of the lacZ gene of Escherichia coli. EMBO J. 1983;2(4):593-7.
Pubmed: 6313347
Sutendra G, Wong S, Fraser ME, Huber RE: Beta-galactosidase (Escherichia coli) has a second catalytically important Mg2+ site. Biochem Biophys Res Commun. 2007 Jan 12;352(2):566-70. doi: 10.1016/j.bbrc.2006.11.061. Epub 2006 Nov 20.
Pubmed: 17126292
Wong LJ, Sheu KF, Lee SL, Frey PA: Galactose-1-phosphate uridylyltransferase: isolation and properties of a uridylyl-enzyme intermediate. Biochemistry. 1977 Mar 8;16(5):1010-6. doi: 10.1021/bi00624a032.
Pubmed: 321007
Lemaire HG, Muller-Hill B: Nucleotide sequences of the gal E gene and the gal T gene of E. coli. Nucleic Acids Res. 1986 Oct 10;14(19):7705-11. doi: 10.1093/nar/14.19.7705.
Pubmed: 3022232
Ujwal ML, Jung H, Bibi E, Manoil C, Altenbach C, Hubbell WL, Kaback HR: Membrane topology of helices VII and XI in the lactose permease of Escherichia coli studied by lacY-phoA fusion analysis and site-directed spectroscopy. Biochemistry. 1995 Nov 14;34(45):14909-17. doi: 10.1021/bi00045a036.
Pubmed: 7578103
Buchel DE, Gronenborn B, Muller-Hill B: Sequence of the lactose permease gene. Nature. 1980 Feb 7;283(5747):541-5. doi: 10.1038/283541a0.
Pubmed: 6444453
Hayashi K, Morooka N, Yamamoto Y, Fujita K, Isono K, Choi S, Ohtsubo E, Baba T, Wanner BL, Mori H, Horiuchi T: Highly accurate genome sequences of Escherichia coli K-12 strains MG1655 and W3110. Mol Syst Biol. 2006;2:2006.0007. doi: 10.1038/msb4100049. Epub 2006 Feb 21.
Pubmed: 16738553
Daley DO, Rapp M, Granseth E, Melen K, Drew D, von Heijne G: Global topology analysis of the Escherichia coli inner membrane proteome. Science. 2005 May 27;308(5726):1321-3. doi: 10.1126/science.1109730.
Pubmed: 15919996
De Reuse H, Danchin A: The ptsH, ptsI, and crr genes of the Escherichia coli phosphoenolpyruvate-dependent phosphotransferase system: a complex operon with several modes of transcription. J Bacteriol. 1988 Sep;170(9):3827-37. doi: 10.1128/jb.170.9.3827-3837.1988.
Pubmed: 2457575
De Reuse H, Roy A, Danchin A: Analysis of the ptsH-ptsI-crr region in Escherichia coli K-12: nucleotide sequence of the ptsH gene. Gene. 1985;35(1-2):199-207. doi: 10.1016/0378-1119(85)90172-6.
Pubmed: 2411636
Saffen DW, Presper KA, Doering TL, Roseman S: Sugar transport by the bacterial phosphotransferase system. Molecular cloning and structural analysis of the Escherichia coli ptsH, ptsI, and crr genes. J Biol Chem. 1987 Nov 25;262(33):16241-53.
Pubmed: 2960675
This pathway was propagated using PathWhiz -
Pon, A. et al. Pathways with PathWhiz (2015) Nucleic Acids Res. 43(Web Server issue): W552–W559.
Propagated from SMP0000903
Highlighted elements will appear in red.
Highlight Compounds
Highlight Proteins
Enter relative concentration values (without units). Elements will be highlighted in a color gradient where red = lowest concentration and green = highest concentration. For the best results, view the pathway in Black and White.
Visualize Compound Data
Visualize Protein Data
Downloads
Settings