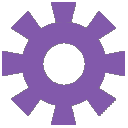
Browsing Pathways
Showing 354121 -
354130 of 605359 pathways
PathBank ID | Pathway Name and Description | Pathway Class | Chemical Compounds | Proteins |
---|---|---|---|---|
SMP0387387![]() |
Ornithine MetabolismEscherichia coli HS
In the ornithine biosynthesis pathway of E. coli, L-glutamate is acetylated to N-acetylglutamate by the enzyme N-acetylglutamate synthase, encoded by the argA gene. The acetyl donor for this reaction is acetyl-CoA. N-acetylglutamic acid is then phosphorylated via an ATP driven acetylglutamate kinase which yields a N-acetyl-L-glutamyl 5-phosphate. The product undergoes a NADPH dependent reduction resulting in N-acetyl-L-glutamate 5-semialdehyde which then reacts with L-glutamic acid through a acetylornithine aminotransferase / N-succinyldiaminopimelate aminotransferase to produce a N-acetylornithine. Deacetylated through an acetylornithine deacetylase, N-acetylornithine finally yields an ornithine. Ornithine interacts with hydrogen ion through an ornithine decarboxylase resulting in a carbon dioxide release and a putrescine. Putrescine can be metabolized by reaction with either l-glutamic acid or oxoglutaric acid. If putrescine reacts with L-glutamic acid, it reacts through an ATP mediated gamma-glutamylputrescine producing a hydrogen ion, ADP, phosphate and gamma-glutamyl-L-putrescine. This compound is reduced by interacting with oxygen, water and a gamma-glutamylputrescine oxidoreductase resulting in ammonium, hydrogen peroxide and 4-gamma-glutamylamino butanal. The previous product is then dehydrogenated through a NADP mediated reaction lead by gamma-glutamyl-gamma-aminobutaryaldehyde dehydrogenase resulting in hydrogen ion, NADPH and 4-glutamylamino butanoate. In turn, the latter compound reacts with water through a gamma-glutamyl-gamma-aminobutyrate hydrolase resulting in L-glutamic acid and Gamma aminobutyric acid. On the other hand, if putrescine reacts with oxoglutaric acid through a putrescine aminotransferase, it results in L-glutamic acid, and a 4-aminobutyraldehyde. 4-aminobutyraldehyde reacts with water through a NAD dependent gamma aminobutyraldehyde dehydrogenase resulting in hydrogen ion, NADH and gamma-aminobutyric acid. Gamma Aaminobutyric acid reacts with oxoglutaric acid through 4-aminobutyrate aminotransferase resulting in L-glutamic acid and succinic acid semialdehyde. Succinic acid semialdehyde in turn can react with with either NADP or NAD to result in the production of succinic acid through succinate-semialdehyde dehydrogenase or aldehyde dehydrogenase-like protein yneI respectively. Succinic acid can then be integrated in the TCA cycle.
|
Metabolite
Metabolic
|
|
|
SMP0387349![]() |
Aspartate MetabolismEscherichia coli O157:H7 str. Sakai
Aspartate is synthesized from and broken down to oxaloacetate, a TCA cycle intermediate, via a reversible transamination reaction with glutamate. This reaction is catalyzed by the aminotransferase AspC or TyrB. Aspartate is a component of proteins and is involved in many biosyntheses pathways like NAD biosynthesis and beta-alanine metabolism. Aspartate can also be synthesized from fumaric acid through an aspartate ammonia lyase. Aspartate also participates in the synthesis of L-asparagine through two different methods, either through aspartate ammonia ligase or asparagine synthetase B. Aspartate is also a precursor of fumaric acid. Again it has two possible ways of synthesizing it. First set of reactions follows an adenylo succinate synthetase that yields adenylsuccinic acid and then adenylosuccinate lyase in turns leads to fumaric acid. The second way is through argininosuccinate synthase that yields argininosuccinic acid and then argininosuccinate lyase in turns leads to fumaric acid.
|
Metabolite
Metabolic
|
|
|
SMP0387393![]() |
Proline MetabolismEscherichia coli HS
The creation of L-proline in E. coli starts with L-glutamic acid being phosphorylated through an ATP driven glutamate 5-kinase resulting in a L-glutamic acid 5-phosphate. This compound is then reduced through an NADPH driven gamma glutamyl phosphate reductase resulting in the release of a phosphate, an NADP and a L-glutamic gamma-semialdehyde. L-glutamic gamma-semialdehyde is dehydrated spontaneously, resulting in a release of water,hydrogen ion and 1-Pyrroline-5-carboxylic acid. The latter compound is reduced by an NADPH driven pyrroline-5-carboxylate reductase which is then reduced to L-proline. L-proline works as a repressor of the pyrroline-5-carboxylate reductase enzyme and glutamate 5-kinase. Three genetic loci, proA, proB and proC control the biosynthesis of L-proline in E. coli.The pathway begins with a reaction that is catalyzed by γ-glutamyl kinase, which is encoded by proB. Next, NADPH-dependent reduction of γ-glutamyl phosphate to glutamate-5-semialdehyde, occurs through catalyzation by glutamate-5-semialdehyde dehydrogenase, encoded by proA. Following this, both enzymes join together in a multimeric bi-functional enzyme complex called γ-glutamyl kinase-GP-reductase multienzyme complex. This formation is thought to protect the highly labile glutamyl phosphate from the antagonistic nucleophilic and aqueous environment found in the cell. Finally, NADPH-dependent pyrroline-5-carboxylate reductase encoded by proC catalyzes the reduction of pyrroline 5-carboxylate into L-proline. Proline is metabolized in E. coli by returning to the form of L-glutamate, which is then degraded to α-ketoglutarate,which serves as an intermediary of the TCA cycle. Interestingly enough, L-glutamate, the obligate intermediate of the proline degradation pathway, is not able to serve as an outright source of carbon and energy for E. coli, because the rate at which glutamate transport supplies exogenous glutamate is not adequate. The process by which proline is turned into L-glutamate starts with L-proline interacting with ubiquinone through a bifunctional protein putA resulting in an ubiquinol, a hydrogen ion and a 1-pyrroline-5-carboxylic acid. The latter compound is then hydrated spontaneously resulting in a L-glutamic gamma-semialdehyde. This compound is then processed by interacting with water through an NAD driven bifunctional protein putA resulting in a hydrogen ion, NADH and L-glutamic acid.
|
Metabolite
Metabolic
|
||
SMP0387370![]() |
Nitrogen MetabolismEscherichia coli E24377A
Nitrogen and nitrogen cycle play an important role in biological process for many microorganisms as catalyzing different reactions. For example, nitrate reduction is used for conversion into ammonia and denitrification, where denitrification is an important cellular respiration process. Nitrogenase enzyme in prokaryotes can fix the atmospheric nitrogen by catalyzing nitrogen fixation (i.e. reduction of nitrogen to ammonia). Nitrate can be introduced into the cytoplasm through a nitrate:nitrite antiporter NarK or a nitrate/nitrite transporter NarU. Nitrate is then reduced by a nitrate reductase resulting in the release of water, an acceptor, and a nitrite. Nitrite can also be introduced into the cytoplasm through a nitrate:nitrite antiporter NarK. Nitrite can be reduced by an NADPH-dependent nitrite reductase resulting in water, NAD, and ammonia. Nitrite can interact with a hydrogen ion and ferrocytochrome c through a cytochrome c-552 ferricytochrome resulting in the release of ferricytochrome c, water, and ammonia. Another process by which ammonia is produced is by a reversible reaction of hydroxylamine with a reduced acceptor through a hydroxylamine reductase. This results in an acceptor, water, and ammonia. Water and carbon dioxide react through a carbonate dehydratase resulting in carbamic acid. This compound reacts spontaneously with hydrogen ion resulting in the release of carbon dioxide and ammonia. Carbon dioxide can interact with water through a carbonic anhydrase resulting in hydrogen carbonate. This compound interacts with cyanate and hydrogen ion through a cyanate hydratase resulting in a carbamic acid. Ammonia can be metabolized by reacting with L-glutamine and ATP-driven glutamine synthetase resulting in ADP, phosphate, and L-glutamine. The latter compound reacts with oxoglutaric acid and hydrogen ion through an NADPH-dependent glutamate synthase resulting in the release of NADP and L-glutamic acid. L-Glutamic acid reacts with water through an NADP-specific glutamate dehydrogenase resulting in the release of oxoglutaric acid, NADPH, hydrogen ion, and ammonia.
|
Metabolite
Metabolic
|
|
|
SMP0387361![]() |
Proline MetabolismEscherichia coli 536
The creation of L-proline in E. coli starts with L-glutamic acid being phosphorylated through an ATP driven glutamate 5-kinase resulting in a L-glutamic acid 5-phosphate. This compound is then reduced through an NADPH driven gamma glutamyl phosphate reductase resulting in the release of a phosphate, an NADP and a L-glutamic gamma-semialdehyde. L-glutamic gamma-semialdehyde is dehydrated spontaneously, resulting in a release of water,hydrogen ion and 1-Pyrroline-5-carboxylic acid. The latter compound is reduced by an NADPH driven pyrroline-5-carboxylate reductase which is then reduced to L-proline. L-proline works as a repressor of the pyrroline-5-carboxylate reductase enzyme and glutamate 5-kinase. Three genetic loci, proA, proB and proC control the biosynthesis of L-proline in E. coli.The pathway begins with a reaction that is catalyzed by γ-glutamyl kinase, which is encoded by proB. Next, NADPH-dependent reduction of γ-glutamyl phosphate to glutamate-5-semialdehyde, occurs through catalyzation by glutamate-5-semialdehyde dehydrogenase, encoded by proA. Following this, both enzymes join together in a multimeric bi-functional enzyme complex called γ-glutamyl kinase-GP-reductase multienzyme complex. This formation is thought to protect the highly labile glutamyl phosphate from the antagonistic nucleophilic and aqueous environment found in the cell. Finally, NADPH-dependent pyrroline-5-carboxylate reductase encoded by proC catalyzes the reduction of pyrroline 5-carboxylate into L-proline. Proline is metabolized in E. coli by returning to the form of L-glutamate, which is then degraded to α-ketoglutarate,which serves as an intermediary of the TCA cycle. Interestingly enough, L-glutamate, the obligate intermediate of the proline degradation pathway, is not able to serve as an outright source of carbon and energy for E. coli, because the rate at which glutamate transport supplies exogenous glutamate is not adequate. The process by which proline is turned into L-glutamate starts with L-proline interacting with ubiquinone through a bifunctional protein putA resulting in an ubiquinol, a hydrogen ion and a 1-pyrroline-5-carboxylic acid. The latter compound is then hydrated spontaneously resulting in a L-glutamic gamma-semialdehyde. This compound is then processed by interacting with water through an NAD driven bifunctional protein putA resulting in a hydrogen ion, NADH and L-glutamic acid.
|
Metabolite
Metabolic
|
||
SMP0281020 |
Cardiolipin Biosynthesis CL(16:0/18:4(6Z,9Z,12Z,15Z)/14:0/18:2(9Z,12Z))Mus musculus
Cardiolipin (CL) is an important component of the inner mitochondrial membrane where it constitutes about 20% of the total lipid composition. It is essential for the optimal function of numerous enzymes that are involved in mitochondrial energy metabolism (Wikipedia). Cardiolipin biosynthesis occurs mainly in the mitochondria, but there also exists an alternative synthesis route for CDP-diacylglycerol that takes place in the endoplasmic reticulum. This second route may supplement this pathway. All membrane-localized enzymes are coloured dark green in the image. First, dihydroxyacetone phosphate (or glycerone phosphate) from glycolysis is used by the cytosolic enzyme glycerol-3-phosphate dehydrogenase [NAD(+)] to synthesize sn-glycerol 3-phosphate. Second, the mitochondrial outer membrane enzyme glycerol-3-phosphate acyltransferase esterifies an acyl-group to the sn-1 position of sn-glycerol 3-phosphate to form 1-acyl-sn-glycerol 3-phosphate (lysophosphatidic acid or LPA). Third, the enzyme 1-acyl-sn-glycerol-3-phosphate acyltransferase converts LPA into phosphatidic acid (PA or 1,2-diacyl-sn-glycerol 3-phosphate) by esterifying an acyl-group to the sn-2 position of the glycerol backbone. PA is then transferred to the inner mitochondrial membrane to continue cardiolipin synthesis. Fourth, magnesium-dependent phosphatidate cytidylyltransferase catalyzes the conversion of PA into CDP-diacylglycerol. Fifth, CDP-diacylglycerol--glycerol-3-phosphate 3-phosphatidyltransferase synthesizes phosphatidylglycerophosphate (PGP). Sixth, phosphatidylglycerophosphatase and protein-tyrosine phosphatase dephosphorylates PGP to form phosphatidylglycerol (PG). Last, cardiolipin synthase catalyzes the synthesis of cardiolipin by transferring a phosphatidyl group from a second CDP-diacylglycerol to PG. It requires a divalent metal cation cofactor.
|
Metabolite
Metabolic
|
|
|
SMP0387354![]() |
Proline MetabolismEscherichia coli O157:H7 str. Sakai
The creation of L-proline in E. coli starts with L-glutamic acid being phosphorylated through an ATP driven glutamate 5-kinase resulting in a L-glutamic acid 5-phosphate. This compound is then reduced through an NADPH driven gamma glutamyl phosphate reductase resulting in the release of a phosphate, an NADP and a L-glutamic gamma-semialdehyde. L-glutamic gamma-semialdehyde is dehydrated spontaneously, resulting in a release of water,hydrogen ion and 1-Pyrroline-5-carboxylic acid. The latter compound is reduced by an NADPH driven pyrroline-5-carboxylate reductase which is then reduced to L-proline. L-proline works as a repressor of the pyrroline-5-carboxylate reductase enzyme and glutamate 5-kinase. Three genetic loci, proA, proB and proC control the biosynthesis of L-proline in E. coli.The pathway begins with a reaction that is catalyzed by γ-glutamyl kinase, which is encoded by proB. Next, NADPH-dependent reduction of γ-glutamyl phosphate to glutamate-5-semialdehyde, occurs through catalyzation by glutamate-5-semialdehyde dehydrogenase, encoded by proA. Following this, both enzymes join together in a multimeric bi-functional enzyme complex called γ-glutamyl kinase-GP-reductase multienzyme complex. This formation is thought to protect the highly labile glutamyl phosphate from the antagonistic nucleophilic and aqueous environment found in the cell. Finally, NADPH-dependent pyrroline-5-carboxylate reductase encoded by proC catalyzes the reduction of pyrroline 5-carboxylate into L-proline. Proline is metabolized in E. coli by returning to the form of L-glutamate, which is then degraded to α-ketoglutarate,which serves as an intermediary of the TCA cycle. Interestingly enough, L-glutamate, the obligate intermediate of the proline degradation pathway, is not able to serve as an outright source of carbon and energy for E. coli, because the rate at which glutamate transport supplies exogenous glutamate is not adequate. The process by which proline is turned into L-glutamate starts with L-proline interacting with ubiquinone through a bifunctional protein putA resulting in an ubiquinol, a hydrogen ion and a 1-pyrroline-5-carboxylic acid. The latter compound is then hydrated spontaneously resulting in a L-glutamic gamma-semialdehyde. This compound is then processed by interacting with water through an NAD driven bifunctional protein putA resulting in a hydrogen ion, NADH and L-glutamic acid.
|
Metabolite
Metabolic
|
||
SMP0281070 |
Cardiolipin Biosynthesis CL(16:0/18:4(6Z,9Z,12Z,15Z)/18:0/22:6(4Z,7Z,10Z,13Z,16Z,19Z))Mus musculus
Cardiolipin (CL) is an important component of the inner mitochondrial membrane where it constitutes about 20% of the total lipid composition. It is essential for the optimal function of numerous enzymes that are involved in mitochondrial energy metabolism (Wikipedia). Cardiolipin biosynthesis occurs mainly in the mitochondria, but there also exists an alternative synthesis route for CDP-diacylglycerol that takes place in the endoplasmic reticulum. This second route may supplement this pathway. All membrane-localized enzymes are coloured dark green in the image. First, dihydroxyacetone phosphate (or glycerone phosphate) from glycolysis is used by the cytosolic enzyme glycerol-3-phosphate dehydrogenase [NAD(+)] to synthesize sn-glycerol 3-phosphate. Second, the mitochondrial outer membrane enzyme glycerol-3-phosphate acyltransferase esterifies an acyl-group to the sn-1 position of sn-glycerol 3-phosphate to form 1-acyl-sn-glycerol 3-phosphate (lysophosphatidic acid or LPA). Third, the enzyme 1-acyl-sn-glycerol-3-phosphate acyltransferase converts LPA into phosphatidic acid (PA or 1,2-diacyl-sn-glycerol 3-phosphate) by esterifying an acyl-group to the sn-2 position of the glycerol backbone. PA is then transferred to the inner mitochondrial membrane to continue cardiolipin synthesis. Fourth, magnesium-dependent phosphatidate cytidylyltransferase catalyzes the conversion of PA into CDP-diacylglycerol. Fifth, CDP-diacylglycerol--glycerol-3-phosphate 3-phosphatidyltransferase synthesizes phosphatidylglycerophosphate (PGP). Sixth, phosphatidylglycerophosphatase and protein-tyrosine phosphatase dephosphorylates PGP to form phosphatidylglycerol (PG). Last, cardiolipin synthase catalyzes the synthesis of cardiolipin by transferring a phosphatidyl group from a second CDP-diacylglycerol to PG. It requires a divalent metal cation cofactor.
|
Metabolite
Metabolic
|
|
|
SMP0388189![]() |
Cardiolipin Biosynthesis CL(a-13:0/i-14:0/18:0/i-20:0)Homo sapiens
Cardiolipin (CL) is an important component of the inner mitochondrial membrane where it constitutes about 20% of the total lipid composition. It is essential for the optimal function of numerous enzymes that are involved in mitochondrial energy metabolism (Wikipedia). Cardiolipin biosynthesis occurs mainly in the mitochondria, but there also exists an alternative synthesis route for CDP-diacylglycerol that takes place in the endoplasmic reticulum. This second route may supplement this pathway. All membrane-localized enzymes are coloured dark green in the image. First, dihydroxyacetone phosphate (or glycerone phosphate) from glycolysis is used by the cytosolic enzyme glycerol-3-phosphate dehydrogenase [NAD(+)] to synthesize sn-glycerol 3-phosphate. Second, the mitochondrial outer membrane enzyme glycerol-3-phosphate acyltransferase esterifies an acyl-group to the sn-1 position of sn-glycerol 3-phosphate to form 1-acyl-sn-glycerol 3-phosphate (lysophosphatidic acid or LPA). Third, the enzyme 1-acyl-sn-glycerol-3-phosphate acyltransferase converts LPA into phosphatidic acid (PA or 1,2-diacyl-sn-glycerol 3-phosphate) by esterifying an acyl-group to the sn-2 position of the glycerol backbone. PA is then transferred to the inner mitochondrial membrane to continue cardiolipin synthesis. Fourth, magnesium-dependent phosphatidate cytidylyltransferase catalyzes the conversion of PA into CDP-diacylglycerol. Fifth, CDP-diacylglycerol--glycerol-3-phosphate 3-phosphatidyltransferase synthesizes phosphatidylglycerophosphate (PGP). Sixth, phosphatidylglycerophosphatase and protein-tyrosine phosphatase dephosphorylates PGP to form phosphatidylglycerol (PG). Last, cardiolipin synthase catalyzes the synthesis of cardiolipin by transferring a phosphatidyl group from a second CDP-diacylglycerol to PG. It requires a divalent metal cation cofactor.
|
Metabolite
Metabolic
|
|
|
SMP0384768![]() |
Nitrogen MetabolismBacteroides sp. 1_1_30
Nitrogen and nitrogen cycle play an important role in biological process for many microorganisms as catalyzing different reactions. For example, nitrate reduction is used for conversion into ammonia and denitrification, where denitrification is an important cellular respiration process. Nitrogenase enzyme in prokaryotes can fix the atmospheric nitrogen by catalyzing nitrogen fixation (i.e. reduction of nitrogen to ammonia). Nitrate can be introduced into the cytoplasm through a nitrate:nitrite antiporter NarK or a nitrate/nitrite transporter NarU. Nitrate is then reduced by a nitrate reductase resulting in the release of water, an acceptor, and a nitrite. Nitrite can also be introduced into the cytoplasm through a nitrate:nitrite antiporter NarK. Nitrite can be reduced by an NADPH-dependent nitrite reductase resulting in water, NAD, and ammonia. Nitrite can interact with a hydrogen ion and ferrocytochrome c through a cytochrome c-552 ferricytochrome resulting in the release of ferricytochrome c, water, and ammonia. Another process by which ammonia is produced is by a reversible reaction of hydroxylamine with a reduced acceptor through a hydroxylamine reductase. This results in an acceptor, water, and ammonia. Water and carbon dioxide react through a carbonate dehydratase resulting in carbamic acid. This compound reacts spontaneously with hydrogen ion resulting in the release of carbon dioxide and ammonia. Carbon dioxide can interact with water through a carbonic anhydrase resulting in hydrogen carbonate. This compound interacts with cyanate and hydrogen ion through a cyanate hydratase resulting in a carbamic acid. Ammonia can be metabolized by reacting with L-glutamine and ATP-driven glutamine synthetase resulting in ADP, phosphate, and L-glutamine. The latter compound reacts with oxoglutaric acid and hydrogen ion through an NADPH-dependent glutamate synthase resulting in the release of NADP and L-glutamic acid. L-Glutamic acid reacts with water through an NADP-specific glutamate dehydrogenase resulting in the release of oxoglutaric acid, NADPH, hydrogen ion, and ammonia.
|
Metabolite
Metabolic
|
|
Showing 354121 -
354130 of 363152 pathways